On the reddish, glittery mud the Inca used for perfecting their stone masonry
Corresponding Author
Email: helmut.tributsch@alice.it
Affiliation
Helmut Tributsch
Carinthia University for Applied Sciences, Bio-mimetics program, Europastrasse 4, 9524 Villach, Austria, helmut.tributsch@alice.it, www.helmut-tributsch.it
Retired from: Free University Berlin, Fachbereich Biologie, Chemie, Pharmazie, Takustr. 3, 14195 Berlin, Germany.
Article Reviewed By:
D W Shiers(Denis.Shiers@csiro.au)
Citation
Helmut Tributsch, On the reddish, glittery mud the Inca used for perfecting their stone masonry(2017)SDRP Journal of Earth Sciences & Environmental Studies 3(1)
Abstract
Due to its impressive appearance, Inca masonry, which mostly consists of volcanic, silica containing rock material, has received much attention. A high level of understanding has consequently been reached of the diverse working steps and tools applied. An exception is the reddish mud, “llancac alpa” in the quechua language, and the “gold”, mentioned by early chroniclers as mortar which fitted the stones and later disappeared. Such techniques were related to folklore and not taken seriously. This study tries to understand them and the question was asked: did Inca builders have access to very acid mud? They did, and used the acid mud from their mines, which generated sulphuric acid through bacterial oxidation of pyrite (fools gold). It reaches an acidity of up to pH = 0.5, which is 104 times more acid than humic acid which is known to weather silica containing rocks via silica gel to the clay mineral kaolin. This acid mud allowed dissolving and softening the rock material superficially to a viscoelastic silica gel. The process could be further enhanced more than tenfold by addition of (oxalic acid containing) plant sap, a skill suggested from popular tradition. In special cases moderate heating of crushed pyrite in gaps between chiselled stones generated additional hot sulphuric acid. Where the stone to stone contact transmitted weight, pressure dissolution in the acidic environment removed material, and silica precipitation regenerated material in cracks and pores elsewhere. It is attempted to reconstruct how the Inca builders applied the silica gel technology for shaping stones, for polishing and fitting them. The appearance of shiny and glassy Inca stone junctions and interfaces is explained via solidification of in-situ generated or additionally added silica gel. Modern processes for conservation of stone monuments against environmental deterioration have independently developed similar silica gel based technology.
Key words: Inca masonry; surface treatment; silicate dissolution; acid mine water; bacterial leaching; stone restoration; pyrite
Introduction
1.1. Inca Stone masonry
Since its discovery by the Spanish conquerors the masonry of royal Inca buildings, which frequently is megalithic and with a fitting which is so exact, that a thin blade can seldom be inserted into a crack between adjacent stone blocks, has been staggering the imagination of visitors and scientists. The Inca art of fitting stones is widely considered one of the most astonishing technical esthetical accomplishments of stone workmanship which deserves the more attention, since iron tools were not known to the Inca civilization. Much of the respect for the Inca culture stems from its impressive architecture and inducing respect was apparently also the motivation for developing this masonry technique. Since the conquest by the Spaniards the highly elaborated Inca stone masonry has generated admiration and stimulated many attempts to understand the techniques, which had been applied (e.g. (Cieza de León, 1553) (Garcilaso de la Vega, 1609) (Watkins,1990)). Nevertheless, today many researchers concerned with ancient Peru agree in the opinion that there is no secret formula beyond a primitive working technique based on stone tools for hammering, polishing with fine sand and an incredible amount of patience and diligence (Mason,1979) (Thompson,1980) (Protzen,1986,1993).
When analysing the stonework done more in detail it has to be considered that its nature and quality is highly variable. There exist polygonal masonry with smaller blocks, cyclopean polygonal masonry with large blocks, encased coursed and sedimentary coursed masonry. In the latter two cases the stones are either not aligned or laid out in horizontal rows. Also the level of perfection may vary significantly in dependence of the location and the political and spiritual relevance of the buildings.
Inca masonry of important monuments are well known for the perfect joining of differently shaped stones. Polygonal stones have often convex, pillow-like faces and sunken joints, with edge angles typically in excess of 90°. The Inca builders seem to have enjoyed the effect of irregularity and the play of shadow and light on their walls. But there is also no doubt that such dovetailed stonewalls much better resisted earthquakes.
Inca masonry is also famous for the manufacturing and transport of stone blocks of up to more than 100 tons. The Inca walls sometimes give the impression that the stones have been handled with unexplainable transport and rock softening techniques, which already gave rise to unconventional interpretations. However J. Protzen (Protzen, 1986, 1993) made a careful experimental study and came to the conclusion that the technology for dressing and transporting stones was not very sophisticated. Most jobs were carried out with stone hammers of quartzite and of different size and a lot of patience. On well dressed stones larger pit scars are found in the interior of the faces and small ones towards the rim. According to experimental studies the Inca occasionally also used polishing techniques. However they were not applied on the faces, but in the joints to improve the fitting. Apparently flat stones were used in linear and rotating movement using a slurry of water and soil rich in clay. (Protzen & Nair, 1997, 2000). It has been suggested, that in new built walls the upper stone faces were initially left uncut. New stones were then carved on their bedding face and one lateral faces. The shape of these faces were then cut out on the already set stone which was then fit into the generated concave depressions. The required threedimensional contours were pounded out and adapted by hammering via many trials. Footprints of stones that once occupied the spot can be recognized on dismantled Inca walls (see below).
However fitting a large stone into an irregular stone gap is nevertheless a major challenge and an interesting technique has been suggested using templates (Andrea, 2002). Another explanation was, that the masons suspended the second boulder on scaffolding next to the first one and traced the shape of the first onto it.
Inca walls are typically inclined inwards by 3°-5°, which makes them, together with their indented and interfacially matched stone pattern highly stable and earthquake resistant. Many stones show pecks or percussion marks, coarser in the centre of the face, finer at the rim and junction with the neighbouring stone. They confirm the experimentally supported strategy of shaping stones by pounding them with increasingly smaller hammer-stones (Protzen, 1986, 1993).
It may be that the perfect fitting of uneven rock faces in selected places was not only aimed at demonstrating superiority but also at securing longevity in an earthquake prone territory. During a stronger earthquake the fitted stones are vibrating and dancing around, but most then find their original place again because gravity favours their position with perfectly matched interfaces. Similar indented stone masonry against earthquakes was also used by other civilizations, e.g. Egyptians (Gizeh), Greeks (Delphy), Iranians (Persepolis). By mostly relying on gravity and friction Inca builders however accomplished an impressing technical standard.
The Inca builders used different rock materials. The rock mineral quality, which the Inca worked for the fabrication of their walls varied from location to location depending on the availability of suitable stone quarries. The softest stone used was limestone (CaCO3). It was, for example applied for the foundations of the Sacsayhuaman fortress, but not for the impressing facades (fig. 2), which were of andesite, igneous stones obtained from a quarry 35 km distant. Andesite, an in the region abundant and excellent volcanic rock was frequently used by Inca builders. It contains 57-63% quartz (SiO2) besides of a mixture of Na- and Ca-Feldspars (NaAlSi3O8, CaAl2Si2O8) and additional minerals. It was, for example also used in Cusco and Raqchi. The Diorites (magmatic origin), which have also been used by Inca builders, are largely equivalent with Andesite (volcanic origin), but were formed slower and show larger crystals. The wall with the famous stone with 12 edges in Cusco belonging to the Palace of Inca Roqa was constructed with Diorite.
Granite, as it was used in Machu Picchu (fig. 1), also contains mainly quartz and K-Feldspar (KAlSi3O8) with some additional minerals. Rhyolite (porphyry) is the volcanic equivalent of granite and has smaller crystals. It has been mainly used by Inca builders for the construction of Ollantaytambo (fig. 3) besides of Andesit and Diorite.
All together it can be said that the rock minerals which the Inca builders used for their most prominent walls were of magmatic and volcanic origin and their composition is characterized by a high content of feldspars and quartz.
1.2. Detailed observations on fitted stone blocks
Many interesting details can be learned from studies of fitted stone blocks in Inca walls. First, it has to be noted that when looking at the cross section of such a wall, often only the front (visible) stone junctions show a perfect fit. In the stonework mostly the load-bearing faces are closely fitted throughout the surfaces. Vertical joints are typically fitted only to a depth of few centimetres. The leftover space is filled with small stones. On the rear side of the wall the fitting is in these cases not perfect and often accomplished via a filling material. However there are also places, where the perfect fit extends into the inside and in some cases all the way through to the opposite side (e.g. Sacsahuaman, Sacred plaza in Machu Pichu, Pisac (fig.4)). In many other cases the joints are only on the rim of the stones but go all the way through on the bearing bottom face. Inside, the adjacent stones have nearly no contact. In well constructed walls, such as in Ollantaytambo, the imprint of the entire irregular upper face of the upper stone can be seen matching the contour of the face of the lower stone. Another Inca monument where this is observed is Pisac (fig. 5).
1.3. What did the early chroniclers say about finishing stones?
There are two early chroniclers of the Inca empire and its collapse, who gave comments on the way Inca builders worked and perfected their elaborate masonry: Garcilaso de la Vega (1609) and Cieza de Leon (1553). The first had a Spanish father, but his mother was an Inca princess, a niece of Inca ruler Huayna Capac. Though born illegitimate he enjoyed a very good education both in Quechua and Spanish language and had on-going contact with previously influential Inca personalities until emigrating to Spain at age 20. There he wrote his Inca history from memory, from Spanish documentation and correspondence with Peruvian contacts. Cieza de Leon was a Spanish “conquistador”, who got involved in the wars among Spaniards on the domination of Peru. Though of modest education he became very interested in history and turned out to be a quite reliable chronicler.
Both chroniclers admire Inca buildings and masonry and the big effort developed to fabricate and transport the stones. They confirm that they used hard stone tools to chip and grind stones. The Jesuit priest Barnabè Cobo (1653) reports that the Inca used obsidian working tools for dressing stones and applied large construction teams for cutting and grinding. In addition to these now well understood techniques Garcilaso de la Vega wrote in his 6th book, chapter 1: “..they were of beautifully cut masonry, and each stone was so perfectly fitted to its neighbours that there was no space for mortar. It is true that mortar was used, and it was made of a reddish clay, which they call in their language “llàncac allpa”, sticky clay, which was made into a paste. No trace of mortar remained between the stones, and the Spaniards therefore state they worked without mortar..”. In book 7 he continues: “..Many of them (the stones) are so closely set that the seam is scarcely visible. To lodge them in this way it would have been necessary to lift each stone and lower it many times, for they had no set-square or even a ruler to help them to put it in place and see if it fitted. Neither did they know how to make cranes or pulleys or any other device to help them raise and lower masonry, though the pieces they handled are terrifyingly large..”. Later he continued: “They did not indeed use mortar made of sand and lime, for they were unacquainted with lime. They did however employ a mortar consisting of a paste of sticky reddish clay, which was used to fill up gashes and pits caused in working the stones…”. (Garsilaso de la Vega describes the colour of the mud with “colorado” and I translate it with “reddish”. Other translators (e.g. Livermore, 1966) translate it with “red”. The real Spanish word for red is however “rojo”. The expression “colorado”, in fact means “coloured”, but shows the tendency towards the colour red. In Spanish it is used in context of “turning red”, “going red” or “blushing”, where a real “red” is not obtained. There is a convincing example: Spaniards named the Colorado river in the US after the colour of the table mountains around. Their colour is definitively not red but “reddish”).
Cieza de Leon writes: “ it is said to be certain that in these buildings of (Ollantay)Tambo or others which had that name, molten gold was found instead of mortar in a certain area of the royal palace or the son temple, with which, together with the bitumen, which they apply, the stones remain fitted to each other”, Garcilaso (6th book, chapter1) confirms this statement by Cieca de Leon and further specifies the technique: “ In many of the royal palaces and temples of the sun they poured in molten lead and silver and gold for mortar. Pedro de Cieza also reports this and I am glad to adduce the evidence of Spanish historians in support of what I know”. In two occasions Cieza de Leon, when explaining Inca masonry, explicitly talks of a “bitumen” (Spanish betùn), which is clearly defined as a combustible mineral (see an explanation further below).
Summarizing the main information from both early chroniclers of the Inca empire: both confirm that a mortar or bitumen was used, described to be reddish and sticky by Garsilaso de la Vega, and combustible (bitumen) by Cieza de Leon. In another description, confirmed by both authors, gold (lead and silver) was poured in between stone boulders in certain cases. Strangely nothing remained visible in the joint between stone blocks. And Garsilaso de la Vega confirmed this. He said: “No trace of mortar remained between the stones”.
Informative is, last not least, also the Quechua name for the reddish clay or bitumen used for fitting together the stones, and which left no trace: “llàncac allpa”. “Allpa” has the significance of a brittle earth or powder or a yellowish-gray clay. It is also the name for powdered garlic or crushed, dried vegetable. “llàncac” means “of sticky properties”, it describes something one would not like to touch with the hands, because it interacts with the skin.
Results
2.1. Is there a need to invoke chemical treatment of Inca masonry?
In spite of the quite convincing reconstruction and experimental verification of Inca stone masonry technology (Protzen et al., 1997, 2000), which places claims of chemical treatments of the fitted stones into the realm of folklore some open questions remain:
On numerous volcanic or magmatic, highly silica containing Inca stones a glazed appearance of stone junctions and sometimes of entire stone surfaces is visible, which suggests a special surface treatment. Sites where this is obvious on part of Inca stones are: inside the city of Cusco: the walls of Koricancha and Loreto Street, Sacsayhuaman, Kenko, Tetecaca, Templo de la Luna (or Amaru Machay), Tambo Machay, Puca Pucara, Pisac, Ollantaytambo, Chinchero, Machu Picchu, and Raqchi.
Fig. 6 shows such a glace appearance from the Ollantaytambo site in the stone junctions of the wall of the 4 meters high, six standing monoliths within the unfinished Sun Temple, when looking upside against the sky. Only the joints are highly reflecting and seem to be vitrified. The surface of the monoliths and the connecting junction stones themselves appear only to be hammered. This suggests that there was a special treatment of the joints and an intention behind.
The reflection of light from some stone joints or stone surfaces is significant. Sometimes the apparent layer even refracts and diffracts light what means that it is to some extent transparent for light. On damaged stone surfaces occasionally a distinct layer with very smooth surface is also discernible (e.g. in Sacsayhuaman). The Peruvian Alfredo Gamarra started to study this “vitrifications“ longer time ago. An identification and cataloging of these intriguing stones has been carried on by Alfredo’s son Jesus Gamarra. These efforts and questions around “vitrified” stone surfaces are discussed in (de Jong and Jordan, 2012) where also the diffraction effects can be seen in a video of ‘the Inca Throne’ at Sacsayhuaman. In this blog a valuable survey of different aspects of “vitrified” Inca stone surfaces is given.
The preliminary conclusion from the present study should therefore be that a partial and selective chemical treatment of Inca stone joints and surfaces appears to have occurred. What techniques were applied and did Inca masonry builders have the materials and knowledge to do it?
2.2 Strategy and guiding hypothesis: knowledge transfer from mining industry
What chemistry would be needed for leaching and superficially softening the hard Inca stones of mostly volcanic or magmatic origin?
It is well known that silica minerals, and specifically Feldspars ((KAlSi3O8 – NaAlSi3O8 – CaAl2Si2O8), which originated from acid magmites such as Rhyolite or granite or crystallized from magma, are slowly weathered by humic acids to kaolin (Chinese clay) (Huang and Keller, 1970). One is dealing with a process of partial hydrolysis forming alumosilicates, which consist of submicroscopically small silicate crystals. Important kaolin deposits were formed like that (e.g. in Central Europe: Germany, Czech Republic). Humic acids may reach an acidity of pH 4.5. Their protons replace potassium ions in the silicate mineral structure, which weakens Si-O-Al bonds liberating aluminium hydroxide and silicic acid. The proton concentration is rate limiting for this process. It is also known that certain complexing acids like salicylic and tartaric acids dissolve silicate minerals ten times faster than other acids.
The question to ask is: did Inca stone wall builders have access to highly acid liquid or mud, so that they could accelerate the weathering process by orders of magnitude?
The investigation based on this question produced a positive answer:
It is suggested here that, during their mining activities Inca miners learned to know acid mine water, arising from the oxidation of sulphur rich metal sulphides like FeS2, pyrite or fools gold. Acid mine water is an unavoidable problem of environmental pollution in sulphide containing mines, for example in mines in which copper is produced from copper sulphides in presence of iron sulphide. The aggressive leaching effect of acid mine water on rocks and wooden equipment in mines is readily visible in mine installations and well known to miners.
But what evidence for sulphide mining exists from the Inca territory from pre-Spanish times? The Incas based their metallurgical activities on experience dating back 2000 to 3000 years to pre-Inca civilizations (Lara Monge, 1906)(Lechtman, 1991). They concentrated their own mining interests on the exploitation of essentially four different metals, gold, silver, copper and tin. However they knew and used also lead or platinum. Most of the mines were deposits of sulphides or associated with sulphides. Inca miners could well distinguish between gold and pyrite (fools gold). But they did not know or use metallic iron (Keatinge, 1988). Mining was a government activity and ordinary people had to offer their work as part of their tribute obligation. They were organized for work in mines in a similar way as for constructing masonry for sacral or administrative buildings. So it could happen that workers with mining experience ended up building masonry. This way the notion of the corroding effect of mine water on stones could have reached the construction sites for Inca buildings. While gold and silver was mainly used for representation and rituals, bronze, the alloy of copper and tin became increasingly important for tools and weapons. In addition copper was also alloyed with gold. This way the mining technology for copper gained more and more strategic relevance, so that numerous sulphide mines were operating in the vast Inca dominated territory. And sulphide mines produced acid mine water, especially when sulphur rich minerals (e.g. pyrite) were present. The process is basically activated by autotrophic, acidophyllic, sulphide oxidizing bacteria, which are gaining their energy from sulphide oxidation and are typically present in mine environments.
2.3 The pyrite mud and its properties
Pyrite is a quite stable mineral. But when crashed pyrite (fools gold) crystals in moderately acidic solution are inoculated with sulphide oxidizing bacteria (e.g. Acidothiobazillus ferrooxidans, Leptospirillum ferrooxidans, Acidothiobacillus caldus, compare Fig. 9, left), these bacteria recognize the sulphide as energy source and start oxidizing it gaining energy for carbon dioxide fixation and multiplication. Within days the pH value of the solution may drop to a value between pH = 0.5 and pH=1 while iron sulphate is formed and Jarosites (e.g. KFe3(SO4)2(OH)6) and Fe3+oxide-hydroxides (such as Goethite, Lepidocrocite (FeOOH)) precipitated. The colour of Jarosite and Goethite is yellow-brown to brown, of Lepidocrocite red (compare fig. 9).
The bacteria, using catalytic intermediates, are extracting and using both, sulfide sulfur and Fe2+ as chemical energy sources from pyrite. The overall reaction of bacterial pyrite oxidation is:
4FeS2 + 2H2O +15O2 bacteria-------------à4 Fe3+ + 8SO42- +4H+ (1)
From this formula it can be seen that acid is generated while Fe3+ and sulfate are the final oxidation products. Many details of the complicated interaction of sulfide oxidizing bacteria with pyrite (fig.9, right) during this biological leaching process have been published (Tributsch & Rojas-Chapana, 2000, 2006 )(Rojas-Chapana & Tributsch,1999, 2000, 2004). Acid production is a side product of the bacteria`s strategy to harvest chemical energy from solid pyrite for carbon dioxide fixation at environmental temperatures. During that process crushed metal sulphide particles are transformed into a reddish mud containing oxidized metal complexes (fig. 10).
Due to their mining activities of sulfide minerals, Inca miners knew acid mud since they were regularly confronted with it and saw its effect on stones and structural installations in the mines. Since the Inca administration demanded work service in varying projects as part of people’s obligations, such information must also have reached the construction sites for Inca buildings. With a pH value as low as 0.5 the acid, and thus proton concentration in pyrite oxidation mud is 104 higher than the acid concentration in humic acid, which is known to slowly degrade silica containing rocks such as Rhyolites or Feldspars via silica gel into Kaolin and clay. The question is now, in what form, at what occasions and how was the acid mud applied. There are several relevant questions: the logistics of transport of the acid mud from the mines (or was it already produced locally?), the possible addition of other substances to the pyrite mud and its handling around the stones for conditioning and optimizing their interfaces. Only speculations can, at present time, be made on the first subject. Concerning the addition of other substances to the pyrite mud there is an interesting trace to be followed from popular tradition in the Andean highlands.
2.4. The story of the Pito and his skill in softening rocks with a herb
Very old legends from Peru, collected by the priest Jorge Lira (e.g. Blázquez, 2003) tell that gods once gave people two gifts of valuable herbs. One was “a plant, which, mixed with other components converted the hardest rocks into a soft and formable mass”. This gift was apparently communicated via an Andean woodpecker, Colaptes rupicola, locally called Pito, a bird of the size of a pigeon, that uses to drill a nest hole into quite rocky facades (but also into adobe walls). Doing that he is told to use a herb to soften the rock material. The Inca stone masons are said to have known the secret.
Already the explorer Percy H. Fawcett (1867-1925) and Hiram Bingham (1875-1956), the re-discoverer of Machu Piccu, learned from and reported the strange story of the Andean woodpecker and his “rock softening” plant, which in folklore is occasionally identified with a bush from the high Andean vegetation, Ephedra andina or with a larger flower, Ageratina (Eupatorium) cuzcoensis. Scientific fact is that neither the skill of the Andean woodpecker in using rock softening plant sap nor the rock softening ability of plant sap themselves could be confirmed.
But something may still be true in this legend, that “plant matter mixed to other components soften stones” because it is so deeply rooted in Andean folklore.
And the possible explanation is simple. Witnesses of Inca masonry work may have seen that crushed plant material was added to the reddish clay, the acid pyrite mud (fig. 10), used for treating Inca stone surfaces. And there would have been a reason for doing this: published experimental work shows that silicate mineral dissolution works via a combined action of chemical complexation and acid attack (Barman et al., 1992) and that organic complexation can significantly increase dissolution (Bennett & Casey, 1994). Below pH 5 dissolution of silica containing rocks itself increases with decreasing pH value and thus increasing acidity. Organic complexing acids accelerate this process. When interacting with aluminosilicate minerals organic acids can complex aluminium, and to a lesser degree silica. This decreases their chemical activity. The result is an increase of dissolution rate independent of solubility constraints. Oxalate is especially active because it efficiently complexes aluminium and increases silicate dissolution by a factor of 15 for a concentration of 1 mM oxalate (Drever & Stillings, 1997)(Drever, 2005). Oxalate, , is a very frequently encountered chemical agent in green plants. It is in fact, as calcium oxalate, distributed among all taxonomic levels of photosynthetic organisms and the level of accumulation can be substantial (Franceschi & Nakata, 2005). Examples are spinach, buckwheat, parsley, beets, chart, poppy, beans, fat hen, or rhubarb, amaranth, beets, potatoes, tomatoes, cereal, celery, chicory.
Many Andean plants could have provided oxalate containing juice for addition to acid pyrite mud for treatment on Inca masonry. If this addition really happened during Inca time and was known to some people, and since the hole drilling Andean woodpecker Pito, Colaptes rupicola, also was suspected to use the juice from a plant, this could have been the origin of the widespread myth of a stone softening plant. In fact, if it was applied, the plant juice, with its oxalate content, just supported and enhanced the dissolution of silicate rock via acid pyrite mud by allowing oxalate to form chemical complexes.
2.5. Did Inca masonry workers apply heating?
Cieza de Leòn two times mentioned the use of a “bitumen” for fitting Inca stones together. Bitumen is a combustible mineral. And he mentioned that “molten gold” was used together with it. Since real gold was never found between fitted Inca stones the “gold” of Cieza de Leòn was apparently “fools gold”, pyrite, which has a very similar appearance, and fits to the “reddish” acid pyrite mud, which is in the centre of attention here. But a bitumen and “molten” material suggest a process involving heat turnover. Did Inca masonry builders apply heat?
Pyrite, FeS2, is indeed a fuel, and, when finely grained subject to self-heating. Its temperature can rise from 30 to 90 °C in three hours, 16 times faster than with finely divided coal. The same amount of pyrite powder would rise the temperature three times as much compared to the same amount of coal powder. Many accidental fires have been generated by finely divided pyrite (Bowes, 1952). This is a well known fact in mining environments and in Peru, for example, an always present problem in heaps of the mine Cerro del Pasco. The rate of self-heating, of course, depends on the ability of the system to release heat into the environment. The process requires moisture and proceeds according to the following two subsequent reactions (Burke & Downs, 1938):
FeS2 + 3O2 = FeSO4 + SO2 (2)
followed by the reaction
2SO2 + O2 + 2H2SO4 (3)
Thereby 340,2 kcal/Mole are liberated as heat. Oxidation occurs proportional to the pyrite surface exposed and, at moderate temperatures above ambient temperature, its rate doubles with each 10 °C rise in temperature (Winmill, 1916). It drastically increases further near 330°C, where real ignition starts.
According to Cieza de Leòn it was obviously known to the Inca masonry workers that the mud used was a bitumen, a combustible mineral. When gold was applied and seen together with the bitumen, the “gold” was apparently “fools gold”, larger particles of pyrite. The fact that “molten” gold, lead and silver was mentioned by him and confirmed by Garcilaso de la Vega also suggests that heat was involved in the process. There was steam involved and this gave the impression that the applied material was heated. It simply makes sense. If, in special applications, crushed pyrite (in addition to some other (accidentally contained) sulphide minerals) would have additionally been added to the reddish mud and sufficiently heated, self-oxidation would have started and accelerated itself with increasing temperature. The result would have been hot sulphuric acid (according to equations (2) and (3)). This would have definitively supported and improved the silica gel forming action of the reddish mud in the gaps between hammered stone blocks. Because of the comparatively small amounts of pyrite in the narrow stone gaps in relation to the large volume of the adjacent stone blocks the heating effect of pyrite oxidation on the volcanic or magmatic stone blocks will have remained small, probably within 50 to 300 degrees. Only colour changes may be expected as a consequence within the stone junctions (iron containing rocks would be reddening, which could explain the brown colouration in the stone joints of fig. 7. left). Characteristic high temperature effects and thermal shock (cracks, disintegration) should therefore typically not be seen on Inca stone surfaces. An exception may be the Inca ruins of Chinchero, which were burned down by the followers of Manco Capac II during war activities.
2.6. Application of acid pyrite mud technique during Inca time
Stone workers in the Inca empire may have used plant material for transport and handling the chemically aggressive pyrite mud. This way they may have discovered that plant juice is significantly increasing the ability of the pyrite mud to superficially soften and dissolve hard volcanic or magmatic rock material. Stone workers may have also discovered that heating the acid mud and adding additional crashed pyrite could significantly improve its ability to dissolve silicate rock.
In Inca stonework masonry the load-bearing faces are closely fitted throughout the surfaces (fig. 5). Vertical joints are typically fitted only to a depth of few centimetres. This may indicate that applying load was an important strategy towards obtaining optimized interfaces.
An important phenomenon which Inca builders apparently empirically applied in their wall technology was “pressure dissolution” and “viscous compaction”. These phenomena were studied in some detail in relation to geochemical and geological processes. Pressure dissolution concerns the dissolution of minerals into an aqueous pore fluid at grain- to -grain contacts in presence of high stress. Where pressure between grains builds up the rock material cracks and degrades. It is compacted because material is consequently dissolved and chemicals and particles are transported away to induce new material growth at stress free locations (Rutter, 1976,1983). The so-called Fowler-Yang equations give an adequate description of this phenomenon (Fowler & Yang, 1999). This phenomenon has also been studied with Biotite and Feldspar (Barnett & Kerrich, 1980) and with quartz Arenites, which can serve as appropriate models for the highly quartz containing volcanic and magmatic stone material used in Inca walls. Pressure solution occurs at stressed individual grain contacts. Dissolved quartz then precipitates on free adjacent grains. Pore fluid migrates and porosity is significantly reduced (Angevine & Turcotte, 1983). This is exactly what one would expect to have happened, when looking at smooth, shiny, “vitrified” interfaces or surfaces of Inca stone blocks (Fig. 6, 8)
This gives an idea of one special technique, which could have been applied by Inca masonry workers: Acid mud, possibly heated, could have been placed between two building stones and the weight of the upper one would have gradually levelled the unevenness of the beforehand chiselled and scraped interface and redistributed dissolved silicate particles for fresh crystallization. This would have helped modelling the shape of the weight producing stone onto the lower one (Fig. 5). Such chemically attacked and “softened” surfaces with irregularly distributed gel-type surface layers could also have been treated and modelled subsequently with mechanical tools. A similar, but modified strategy could have been applied to vertical interfaces between stone blocks.
2.7. Evidence for chemical treatment of Inca stone walls
It is obvious that in-situ archaeometric studies of shiny, ”vitrified” layers on worked Inca stones will be needed to further support the concept of the here given material technology. This will need the cooperation with archaeological authorities. Fortunately for the present study one experiment has already been made and published (De Jong & Jordan, 2012): A small sample from the Peruvian Inca site called Tetecaca has been collected from a limestone block showing a smooth overlayer. This smooth layer has been analized by the University of Utrecht, Holland. The surface layer, approximately 10 micrometer thick on top of the limestone (besides of Ca, C, and O showing only low amounts of trace elements) showed high concentrations of Si, Al and Mg. This indicates that a special material layer on silica-basis has been superposed.
If acid mud on the basis of bacterially or chemically oxidized FeS2 was used, iron, Fe, and sulphur, S, from the final products, jarosite, [KFe33+[(OH)6|(SO4)2], and Fe3+ oxide-hydroxides should be detected, even though most of these product could have been washed out. In the mentioned element analysis of a „vitrified“ layer on limestone these elements, Fe and S, actually show up. Of course, more detailed studies are necessary to confirm such findings, but it seems that a silica containing layer has intentionally been superposed onto a a limestone material to make the interface more resistant and perfect. The here given explanation: a silica gel was produced by treating finely pounded volcanic rock material with acid pyrite mud. This resulting gel was then distributed onto or between lime stone interfaces for precipitation and hardening (compare modern silica based stone preservation techniques explained further below).
Discussion
Volcanic and magmatic rocks, as used in Inca masonry, are known to be slowly weathered by humic acids to kaolin and clays. Pyrite mud from mines, which has been oxidized by sulphide oxidizing bacteria can reach acid concentrations 104 times higher, facilitating a correspondingly faster dissolution and formation of superficial silica gel. In addition the pyrite mud could have been heated or oxalate containing plant sap could have been added. This could have increased solubility of silicate rock material by another one to two orders of magnitude. All together this suggests that a chemical dissolution and transformation technique would have been applicable and could provide the basis for explaining the degree of perfection of stone work reached in part of Inca stone masonry. For confirming these ideas and for actually reaching a high level of understanding experimental archaeological research will be nevertheless unavoidable.
It is important here to have again a look at the comments given by the early chroniclers of the Inca civilization, which gave the impulse for this study. The reddish clay, “llàncac allpa”, mentioned by Garcilaso de la Vega (1609), which was made into paste for application, however finally disappeared, is well represented by the acid pyrite mud shown in fig. 9. It has the right brown-reddish colour and macroscopic solid particles (the “allpa”) disappear with growing oxidation (compare formula (1)). Garcilaso de la Vega also explains its application. He speaks of a “paste of sticky red clay, which was used to fill up gashes and pits caused in working the stones…”. This exactly describes how the acid pyrite mud should have been applied.
Also the observations of the informants of Cieza de Leon (1553) seem to make sense. By stating “besides of the commonly used bitumen in certain parts of the palace and the temple of the sun they used instead of mortar also molten gold” he first confirms the “commonly used bitumen or mortar”, which later obviously disappeared. It can only be the reddish clay “llàncac allpa” of Garcilaso de la Vega. Pyrite, contained in it is actually a combustible bitumen. But then he also speaks of molten gold, which was applied (and obviously also disappeared from the joints of Inca stones).
His informants may have seen pyrite particles in the reddish mud poured into or handled in the narrow gaps between stone blocks. Hot acid mud is much more reactive and acid concentration will also have increased due to evaporation of water. Only a relatively moderate local heating to accelerate the dissolution action of sulphuric acid on the silicate rock material or to activate the pyrite oxidation was required (to below 100 °C or below 330°C respectively; the boiling point of sulphuric acid is 338°C). And when the informants looked at what was poured in between stone blocks they may have seen glittering pyrite particles (fig. 9) – fools gold. Most Inca stones are of volcanic or magmatic origin, are quite heat resistant and do not show signs of elevated heat treatment.
Garcilaso de la Vega, while describing the reddish clay, also confirms that “molten lead and silver and gold were poured in for mortar”. Acid pyrite mud from mines may typically contain additional sulphide minerals (e.g. besides of pyrite (FeS2, golden) also Marcasite (FeS2, tin-white to bronze-yellow), Molybdenite (MoS2, lead-silvery-grey), Covellite (CuS, blue), Bornite (Cu5FeS4, copper red to purple)). From occasionally seeing such diverse crystals in the applied reddish mud it may have been concluded that molten lead, silver and gold is poured between the stone blocks.
The effect of the described chemical Inca stone technique for the interface and surface treatment of silicate blocks for optimized masonry is the following: First solid silicate material is broken down in undesired (pressure stressed) location (by acid, complexing agents or thermal pyrite oxidation) and transformed into small liberated particles and a silica gel. Then the silica particles are settling down in desired (unstressed) locations and allowed to solidify again.
Interestingly this empirical Inca silica gel strategy applies similar principles as modern techniques aimed at repairing weathered stones in historic monuments and buildings. The difference is that the silica species leading to the gel are typically not generated from the stone material but supplied from outside. (Zornoza-Indart & López-Arce, 2016)(Zendri et al., 2007)(Aggarwal et al., 2015). Two main strategies are applied. One consists in choosing organic silicide compounds composed of silicic acid and alcohols. They penetrate cracks, dissociate and form amorphous silica particles, a silica gel, which gradually leads to a superficial solidification of the stone material. The other technique applies first a chalk of silica on a damaged surface. Subsequently a silica-organic liquid is applied, which penetrates it, forms colloidal silica and silica gel, which subsequently solidifies.
Also Inca stone masons occasionally applied silica gel prepared separately, probably from finely ground volcanic or magmatic rocks treated with acid pyrite mud. From the above mentioned analysis of a silica based layer on a limestone from the Inca ruins in Tetecaca (De Jong & Jordan, 2012) it can be deduced that actually a silica layer, polluted by some iron and sulphur from the acid pyrite mud, was generated above a lime underground. Modern stone restoration would have yielded the same result.
If the here proposed silica gel based technology can be further confirmed for Inca masonry this would raise the level of their work from “no secret formula beyond a primitive working technique based on stone tools” (Protzen,1986,1993) to an astonishing chemical-technical engineering standard. This would be justified, considering the large expertise with metals and minerals which pre-Inca and Inca mining experts had reached including the processing of platinum with a melting temperature of 1768 °C to ceremonial and utilitarian jewellery,
The author expects that Inca strategies to perfect stone junctions in stone masonry will be better and better understood, when more will be learned about ambient temperature silica technology. This field of knowledge is still poorly developed, but has been applied with great success in biology. Attention is pointed to the large family of diatoms, belonging to light collecting phytoplankton, with their elaborate microscopic silica structures, which, in contrast to our high temperature glass technology, are produced at very low energy cost. Also the elegant structures of glass sponges (e.g. Euplectella aspergillum) in deep sea environments should be mentioned. They apply ambient temperature glass of high optical quality and even elastic glass fibres, made up of concentric thin silica sheets linked by proteins (Aizenberg et al, 2003).
Inca masonry builders may have had esthetical and psychological reasons to aim at such perfection with silicate stone junctions and developed their silica technology empirically. We should learn more about it, should undertake experimental archaeology to understand it properly.
Images and Tables
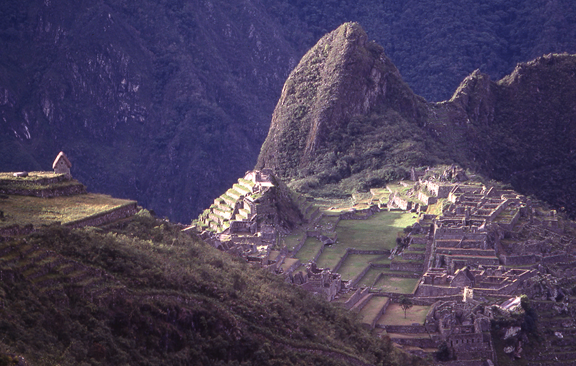
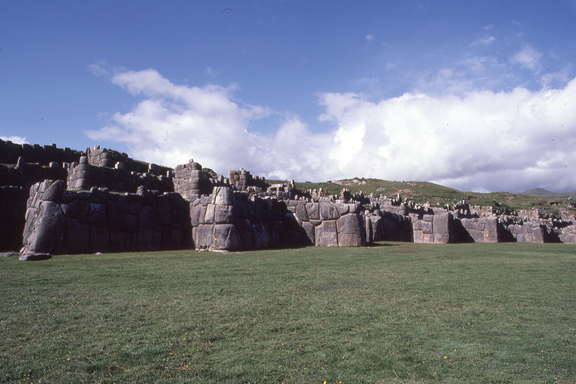
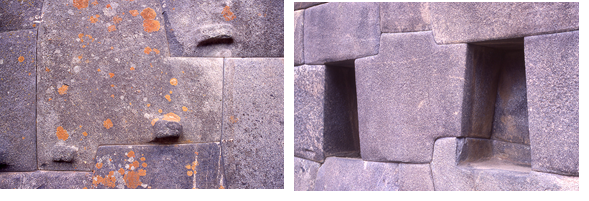
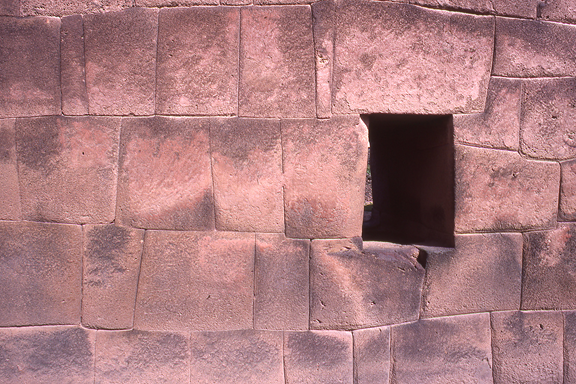
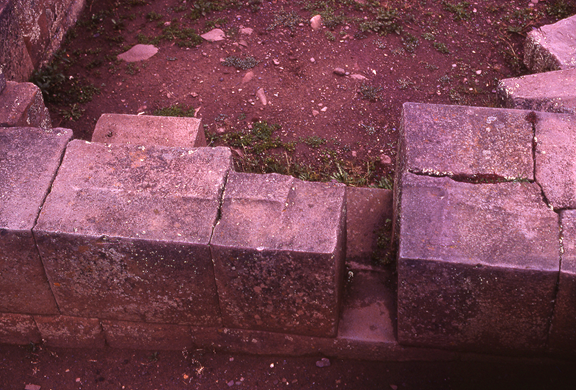
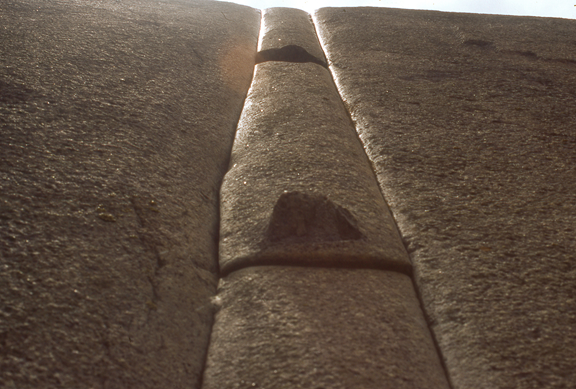
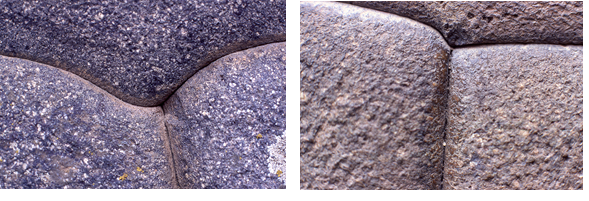
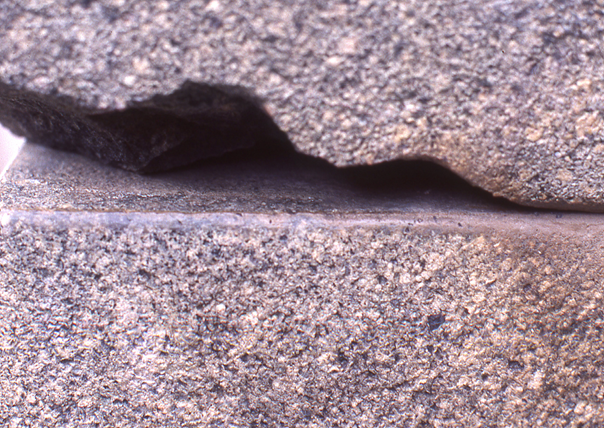
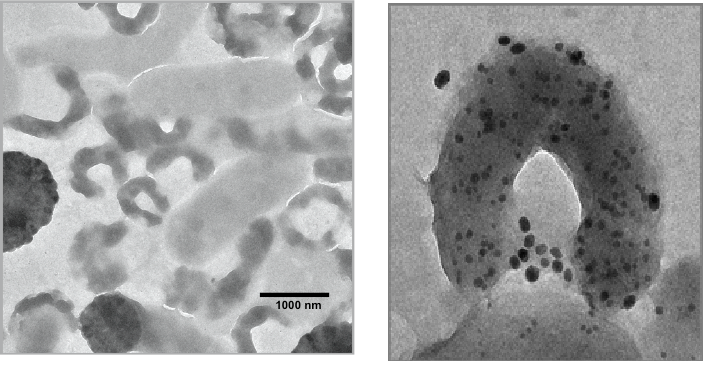
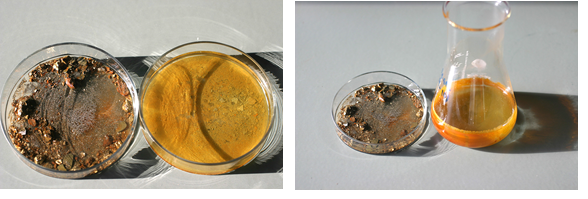
References
Aggarwal, P.; Pratap Singh, R.; Aggarwal, Y. (2015) Use of nano-silica in cement based materials-A review. Cogent Engineering, [2], 1078018. . https://doi.org/10.1080/23311916.2015.1078018
View ArticleAizenberg, J., Sundar, V. C., Yablon, A. J., Weaver, J. C., and Chen,G., (2004), Biological glass fibers: Correlation between optical and structural properties, PNAS 2004 101 (10) 3358-3363; doi:10.1073/pnas.0307843101
View ArticleAndrea, D. (2002), , retrieved, 14/11/ 2016)
View ArticleAngevine C. L. and Turcotte D. L., (1983) Porosity reduction by pressure solution: a theoretical model for quartz arenites, Geol. Soc. Am. Bull., vol. 94, 1129?1134 (1983) 94<1129:PRBPSA>2.0.CO;2
View ArticleBarman A.K., Varadachari C.,Gosh, K, (1992), Weathering of silicates by organic acids I. Nature of cation solubilization, Geoderma 53,45-63 90020-8
View ArticleBarnab? Cobo (1953) Historia del Nuevo mundo, book 14, chapter 12
Barnett, R.L., Kerrich, R., (1980), Nature 283,185-187
View ArticleBennett P.C., Casey W. (1994) Chemistry and Mechanisms of Low-Temperature Dissolution of Silicates by Organic Acids. In: Pittman E.D., Lewan M.D. (eds) Organic Acids in Geological Processes. Springer, Berlin, Heidelberg,
View ArticleDOI
View ArticleBl?zquez, B. H. (2003), Las Piedras de Plastilina, Revista: Vivat Academia 46, (retrieved: 25/11/2017)
View ArticleBowes, P.C., (1952), Note on spontaneous heating and ignition in iron pyrites; Fire research notes 39; (retrieved 29/11/2017
View ArticleBurke,S.P.,Downs, R.,(1938) Trans. Amer. Inst. Min. Met. Eng. 130, 425
Cieza de Le?n, Pedro (1553), La Cr?nica del Per?, Buenos Aires, 1945,; Secunda parte de la Cronica del Peru, Instituto de Studios Peruanos, Lima (1967), chapter 27; Parte Primera de la Cronica del Peru. Edici?n Espasa-Calpe, Madrid 1932, 1962, chapter 44
De Jong Peter & Christopher Jordan, (2012), Evidence of Vitrified Stonework in the Inca Vestiges of Peru, Blog: retrtieved febrary 13, 2016.
View ArticleDrever, J. I. (2005), Surface and ground water, weathering and soils, p. 175
Drever, J. I., L. L. Stillings. (1997). The role of organic acids in mineral weathering. Coll Surf 120:167?181 03720-X
View ArticleFranceschi V.R., Nakata P.A. (2005), Calcium oxalate in plants: formation and function, Annu. Rev. Plant. Biol. 56, 41-71, PMid:15862089
View Article PubMed/NCBIGarcilaso de la Vega (El Inca), (1609) Comentarios Reales de los Incas. 6th book, chapter 1, 7th book, chapter 27; Madrid 1723 or Edition Carlos Aran?bar editor), Fondo de Cultura Econ?mica, Ciudad de M?xico 1991, ISBN 968-16-4892-7; : 2005, ISBN 968-16-4893-5.,
Huang, W.H., Keller, W.D.,(1970) Dissolution of rock-forming silicate minerals in organic acids: simulated first-stage Weathering of fresh mineral surfaces, The Americal Mineralogist, 55, 2076-2094
Keatinge, R.W. (1988). Peruvian Prehistory: An Overview of Pre-Inca and Inca Society. Cambridge University Press. PMid:3384604
PubMed/NCBIFowler A. C. and Yang X. S.,(1999), Pressure solution and viscous compaction in sedimentary basins, J. Geophys. Res., B104, 12898?12997 (1999).
View ArticleLara Monge F. (1906). La metalurgia del oro y la plata en el Peru antiguo, en la colonia y en las culturas Inca y Pre-Incas, I Congreso International de Mineria y Metalurgia en el Contexto de la Historia de la Humanidad: Pasado, Presente y Futuro . Mequinenza 6-9, julio 2006. P-09, pp. 105-116; www.sedpgym.es/descargas/libros_actas/MEQUINENZA_2006/Mequinenza_31.pdf
Lechtman, H. (1991). "The Production of Copper-Arsenic Alloys in the Central Andes: Highland Ores and Coastal Smelters?". Journal of Field Archaeology 18: 43?76. doi:10.1179/009346991791548780.
View ArticleLivermore, H.V., (1966), Royal Commentaries oft the Incas and General History of Peru, by Garsilaso de la Vega, El Inca, University of Texas Press, Austin & London
Mason, J.A., (1979), The Ancient Civilization of Peru, p. 162, 274, Penguin Books, New York 1979 Protzen, J.-P. (l986). Inca stonemasonry. Scientific Amer. 254: 94-105.
Protzen, J.-P. (1993), Inca Architecture and Construction at Ollantaytambo. Oxford University Press. New York. 1993.
Protzen, J.-P. and Nair, S.E. (1997). Who Taught the Inca Stonemasons their Skills? A Comparison of Tihuanaco and Inca Cut-Stone Masonry. Journal of the Society of Architectural Historians: 52:2, 146-167.
View ArticleProtzen, J.-P., Nair, S.E., (2000), "On Reconstructing Tiwanaku Architecture." Journal of the Society of Architectural Historians. September 2000. 358-71.
View ArticleRojas-Chapana J., Tributsch H. (2001), Biochemistry of Sulfur Extraction in Biocorrosion of Pyrite by Thiobacillus ferrooxidans Biohydrometallurgy and the Environment toward the Mining of the 21st Century,Proceedings of International Biohydrometallurgy Symposium IBS-99, Madrid June 1999 Editors: R. Amils and A. Ballester; Elsevier, Process Metallurgy 9A, Part A (1999) 597-605 and Hydrometallurgy 59 (2001) 291-300
Rojas-Chapana J., Tributsch H.,(2000), Bio-Leaching of Pyrite accelerated by Cysteine, Process Biochemistry 35 (8) (2000) 815-824 00142-9
View ArticleRojas-Chapana J., Tributsch H., (2004), Interfacial activity and leaching patterns of Leptospirillum ferrooxidans on pyrite, FEMS Microbiology Ecology 47 (2004) 19-29 00221-6
View ArticleRutter, E. H. (1976). "The kinetics of rock deformation by pressure solution". Philosophical Transactions of the Royal Society A 283: 203?219. doi:10.1098/rsta.1976.0079. JSTOR 74639.
View ArticleRutter, E.H. (1983). "Pressure solution in nature, theory and experiment.". Journal of the Geological Society, London 140 (5): 725?740. doi:10.1144/gsjgs.140.5.0725. Retrieved 24 November 2010.
View ArticleThompson, D.E. (1980), Book Review: Tnca Architecture, Science 210, 779 PMid:17739543
View Article PubMed/NCBITributsch H., Rojas-Chapana J.(2000), Metal Sulfide Semiconductor Electrochemical Mechanisms Induced by Bacterial Activity, Electrochimica Acta, 45 (2000) 4705-4716 00623-X
View ArticleTributsch H., Rojas-Chapana J. (2006), Bacterial Strategies for Obtaining Chemical Energy by Degrading Sulfide Minerals, in: The Practice, Theory and Microbiology of Biomining, Springer Verlag; ed. by Douglas E. Rawlings, D. Barrie Johnson, Chapter 13 (2006) 263-280
Watkins, I. 1990. How Did the Incas Create Such Beautiful Stonemasonry?" in "Rocks and Minerals" Vol. 65 Nov/Dec 1990.
Winmill, T.F., (1916),Trans. Inst. Min. Eng. 51,500
Zendri, E.; Biscontin, G.; Nardini, I.; Rialto, S. (2007) Characterization and reactivity of silicatic consolidants. Constr. Buildi. Mater., [21], 1098?1106.
View ArticleZornoza-Indart, A.; L?pez-Arce, P. (2016) Silica nanoparticles (SiO2): Influence of relative humidity in stone consolidation. J. Cult. Herit., [18], 258?270.
View Article