Ben Salem Zohra
E-mail: bensalemzohra@gmail.com
Spatial pattern of ciliates in relation to environmental factors at Sfax south coast (Gulf of Gabes, Tunisia)
Corresponding Author
Affiliation
Ben Salem Zohraa,b,* and Habib Ayadia
a Biodiversity and Aquatic Ecosystems UR/11ES72 Research Unit, Department of Life Sciences Research, Sfax Faculty of Sciences, University of Sfax, Street of Soukra Km 3.5. BP 1171 - PO Box 3000 Sfax, Tunisia.
b Université de Franche-Comté, Laboratoire de Chrono-Environnement, UMR CNRS 6249 1, Place Leclerc, F-25030 Besançon cedex, France
Citation
Zohra, Spatial pattern of ciliates in relation to environmental factors at Sfax south coast (Gulf of Gabes, Tunisia)(2016)SDRP Journal of Earth Sciences & Environmental Studies 1(2)
Abstract
Data for ciliate abundance, biomass composition, with relevant physicochemical parameters, were studied in the south of Sfax from twenty stations during winter 2008. A total of 18 ciliate taxa representing 10 different genera were identified during the monitoring periods. The Tintinnida group is the most diversified (11 species) accounting for 77% and 92% of the total abundance and biomass, respectively, followed by Choreotrichida and Strombidiida. The ciliate assemblage is dominated by the medium-sized (from 30 µm to 60µm) species and T. beroidea, T. balechi and T. nana are the most abundant. High chlorophyll-a concentration, N/P ratio lower than the Redfield and low Shannon index of ciliate indicated that the water quality of the south coast of Sfax is degraded. So, the coupling of the chemical and biological parameters may serve as good tools to monitor the marine ecosystems.
Introduction
Planktonic ciliates are important components ofmicroplankton communities (1,2). They play a crucial role inthe functioning of microbial food webs as important mediators of energy transfer from low to higher trophic levels (3–5). Owing to their rapid growth and delicate external membranes, ciliates react more quickly to environmental changes than most other eukaryotic organisms (6,7)and the relationships between ciliate sand environmental conditions were increasingly investigated (7–10).
Sfax, the country’s second largest city, is one of the gulf’s main harbors and an important industrial center whose pollution level is in contrast with nearby and beautiful Kerkennah Island (11). The coastal ecosystems are the most threatened biotopes due to anthropogenic pressure and climate change (12). In the south coast of Sfax the main pollution sources are certainly attributable to the two industrial areas of Madagascar and Sidi Salem, the SIAPE and the sewage treatment station ONAS (National Sanitation Office). These areas are known for producing pollutants released from domestic, industrial, mining and agricultural effluents (13–15).We are aiming, through this work, to study the spatial distribution and the ciliate community in relation to physicochemical parameters of the water, on the south coast of Sfax, submitted to high pollution pressure.
Materials & Methods
Study area
This study was carried out in the south coast of Sfax (central eastern coast of Tunisia, between 34°N and 10°E), a city with a dry climate (average precipitation: 210 mm) and by winds, waves and currents (0.2–0.3 s-1) having a predominant north-south direction (16). This area is surrounded by the industrial zone of Sfax, and the Phosphogypsum and Manure Production Factory SIAPE SociétéIndustrielled ’Acide Phosphoriqueetd’ Engrais).
Field sampling
Samples for nutrients ciliate and chlorophyll-a were collected in February 2008 at 20 stations (from 0.5 to 4.2 m in depth) along the coast south of the Sfax City (Fig. 1). Samples for nutrient analyses (250 ml) were preserved immediately upon collection (-20 °C, in the dark). For ciliate analyses, seawater samples (1L) were preserved with Lugol iodine solution (4%) (17) and were stored in the dark at low temperature until enumeration. Water samples (1L), for chlorophyll-a analyses, were filtered by vacuum filtration onto a 0.7-mm pore size and 47-mm-diameter glass fiber filter Whatman GF/F and immediately stored at -20 °C.
Physicochemical analysis
Temperature, pH, salinity and conductivity were measured immediately in the field using a multi parameter kit (Multi 340 i/SET). Nutrients (nitrite: NO2-, nitrate: NO3-, ammonium: NH4+, orthophosphate: PO43- and silicate: Si) were analyzed by a BRAN and LUEBBE type 3 analyzer and concentrations were determined colorimetrically. Suspended matter concentrations were measured using the dry weight of the residue after filtration of 0.5 L of seawater onto Whatman GF/C membrane filters.
Chlorophyll-a and ciliate analysis
Chlorophyll-a concentration was determined by spectrometry, after extraction of the pigments in acetone (90%) and using the SCOR-UNESCO equations (18). Subsamples (50 ml) were enumerated under an inverted microscope, after settling for 24 to 48 h, using the method of Utermöhl(19). Identification of ciliate species was made according to various keys (20–22). Biomass values were calculated using the conversion factor for the ciliate species proposed by Putt and Stoecker(23): 1 pg C = 1 µm3 × 0.19. The level of community structure was assessed with Shannon and Weaver’s (24)H' diversity index.
See Equation 1 in Images & tables below
Where Pi is the proportion of the total count arising from ith species and N is the total cell abundance.
Data analysis
Contour plots were made using Surfer softwar, version 11. Univariate analyses (Spearman's correlation coefficients) were carried out using the statistical program IBM SPSS Statistics 20Package Program to reveal the relationship between the ciliate community (abundance, biomass and different groups of ciliate) and abiotic factors at each sampling station.
Results
Environmental parameters
The water temperatures ranged from 14 to 16.20°C (mean ± s.d. = 15.33 ± 0.64°C) (Fig. 2a). The pH ranged from 6.79 to 8.24 (mean ± s.d. = 7.76 ± 0.44). Salinity ranged from 39.00 to 40.10 psu (mean ± s.d. = 39.78 ± 0.38 psu) (Fig. 2b). The lowest salinity (39psu) was recorded at stations front to effluent point of urban leachate (Fig. 2c). The suspended matter ranged from 44.00 to 84.00 mg l-1 (mean ± s.d. = 53.70 ± 8.21 mg L-1) (Fig. 2d).
The chemical variables analyzed during this study are given in Table 1.Total phosphorus (T-P) concentrations were high (3.62–73.31μmol l-1). Orthophosphate concentrations were lower (0.51–8.52μmol l-1) (Fig. 2e), representing only 13.85% of total phosphorus. Total nitrogen (T-N) concentration varied from 25.78 to 79.27 μmol l-1 (mean ± s.d. = 44.55± 13.28 μmol l-1). The dissolved inorganic form (NO2−+ NO3−+NH4+= DIN) represented 48.6 % of the total nitrogen. Ammonium concentrations accounted for 61.30% of total DIN followed by nitrate 35% and nitrite 3.7%. The Redfield ratio showed an irregular distribution along all stations. DIN/DIP ratio was greater than the Redfield ratio (16) in stations S1, S2, S6, S13 and S14 (Fig. 2f) suggesting potential P limitation and it is lesser than the Redfield ratio in the other stations, which suggests a potential N limitation.
Structural composition of the ciliate community
We found 18 ciliate taxa, representing 10 genera. The order Tintinnida was the most diverse group and accounted for 61% of the total species number, followed by Choreotrichida (22%) and Strombidiida (17%)(Fig. 3a). Ciliates were present with the abundance varying between 4.00 and 523.99 × 102 cells l-1(mean ± SD =121.85 ± 29.91 × 102cells l-1) (Table 1). With regard to the total abundance, the order Tintinnidawas the most abundant group (77%) (Fig. 4a) dueto the dominance of Tintinnopsisberoidea (58%), Strobilidiumneptuni (19) and Tintinnidiumbalechi (8%) (Fig. 3b). The order Choreotrichidaac counted for (20%) of the total abundance, followed by Strombidiida(3%)(Fig. 4a). The maximum ciliate abundance is recorded in the station S16 ascribed to the high densities of different ciliate species including T. cylindrica (34 × 103 cells l-1), S. neptuni (11.1 × 103 cells l-1), T. balechi (2.5 × 102 cells l-1), S. nivalis (2.1 × 102 cells l-1), S. conicum (1.3 ×102 cells l-1), T. nana (800 cells l-1), S. acutum (300 cells l-1), S. capitatum (100 cells l-1) and Pelagostrobilidium sp. (100 cells l-1) (Table 2).
The biomass of the ciliate community ranged between 0.86 and 129.26 μg C l-1 (mean value: 26.18 ± 7.00 μg C l-1) during this study (Table 1). With regard to the total biomass, the order Tintinnida was the most dominant group (92%), followed by Choreotrichida (6%) and Strombidiida(2%) (Fig. 4b).Ciliate community diversity estimated by the Shannon index varied from 0.18 to 0.63 bits cells l-1) (mean ± SD=0.47± 0.12 bits cells l-1). The maximum was recorded at S18, associated with the presence of 9 species (Fig. 5).
The mean length of the different ciliates is given in Table 2. The ciliate community ranged within three size fractions: small (20–30 µm), medium-sized (30–60 µm) and large ciliates (>60 µm), belonging to distinct taxonomic groups and showing different ecological preferences as well. The three size fractions were well represented in the south coast of Sfax waters with the dominance of the medium-sized ciliates (50%) (Fig. 6).Small ciliates (5species) were comprised of different choreotrichs such as Strobilidiumneptuni, Leegaardiella sol, Lohmanniellaoviformis and the tintinnida Tintinnopsisparva and Tintinnopsisparvula. Medium-sized ciliates(9 species) were represented essentially by Tintinnopsis nana, Strombidiumconicum, Tintinnidium balechi and Tintinnopsisberoidea. Large ciliates (4 species) were comprised of Tintinnopsis campanula, Tintinnopsistubulosa, Stenosemellaavellana and Favellaehrenbergii showed the maximum size mainly attributed to its large lorica (length = 139 µm).
Correlations (Spearman, SPSS) between various environmental variables and the total abundance, carbon biomass of the ciliate community and abundances of different ciliate groups are shown in Table 3. The total abundance of the ciliate community exhibited positive correlations with water temperature (r = 0.514, P< 0.01) and chl-a (r = 0.386, P<0.01), and negative correlations with salinity (r = -0.376, P< 0.01) and inorganic nutrients (PO43-, NO2-, NO3- and NH4+). The total carbon biomass exhibited a similar correlation pattern with physicochemical parameters. The order Tintinnida was negatively correlated with salinity (r = -0.283, P<0.05), NO2- (r = -0.138, P<0.05) and PO43- (r = -0.281, P< 0.05). The order Strombidiida and Choreotrichida exhibited positive correlation with Chl-a (r = 0.529, P<0.01) and (r = 0.352, P<0.01), respectively, and negative correlation with all inorganic nutrients.
Discussion
During this study, the recorded values of temperature and salinity were typical of arid to semi-arid zones (9). The lowest value of pH was observed in the southern stations especially in station 17, in front of the outlet effluent of the industrial park, ONAS and the phosphate treatment plant (SIAPE). N/P ratio was widely used as an indicator of nitrogen or phosphorus limitation (25). The high DIP concentrations as well as their high magnitude relative to total phosphorus and the resulting low N/P ratios (< 16 Redfield ratio) in the south coast, N availability was the limiting element for phytoplankton growth and contrasting with results from the gulf of Gabes currently report phosphate depletion (26,27). These results may be explained by the continuous supply of DIP effluent from SIAPE and by the fact of a faster recycling of phosphorous than nitrogen (28). The chlorophyll-a concentrations recorded in present study were higher than those founded in the Gulf of Gabes < 0.50 µg L-1, known as oligotrophic (29, 30). Thus, high chlorophyll-a concentration together with an N/P ratio lower than the Redfield ratio indicate that this ecosystem is under pollution pressure (31).
Spirotrichea was the predominant class in the south coast of Sfax, which is a common feature in many aquatic ecosystems (3,9,32–34). During our study, Tintinnidsac counted for a large proportion (61%) of the ciliate communities. Their abundance seemed to be related to their morphology that they can escape grazing due to the presence of a protective lorica(35), their tolerance of environmental stress and high adaptability to eutrophic environments than other ciliates (36)and their broad trophic diversity (37)which is relatable to the availability of small diatoms and large phytoplankton prey (38,39). The Tintinnida Tintinnopsis genus is common in coastal systems (40,41). In the present study, the most abundant species was Tintinnopsisberoidea, this species is quite common in coastal areas and in particular in gulfs (Gulf of Naples, (41)), (Gulf of Tunis, (32)) and (Gulf of Gabes, (9)). This result suggests that T.beroidea (medium-size = 39 µm) performed better than other species probably owing to more flexible adaptive strategies, to particular environmental conditions (wind mixing, light,..) and the shallowness of the water sampling (9).According to Pérez et al.(42)the copepods preferentially consume small ciliates. Thus, the lowest abundance of the small ciliate, <30 µm, which accounted for about 28% of total biomass may be due to the grazing pressure of mesozoo plankton, such as copepods (9). The diversity index, namely Shannon-Weaver diversity index, can serve as an indicator that the ecosystem is under the influence of pollution stress or eutrophication (43) and higher values for these indices indicate better water quality status (44,45). In the present study, H’ had low values in sampling stations, with mean value of H’ = 0.47 bits cells l-1 indicating that the south coast of Sfax is submitted on degradation of the water quality.
The spearman correlation analyses demonstrated that the spatial variations of ciliate community at the south coast of Sfax, in terms of both abundance and carbon biomass, were positively correlated to water temperature and negatively correlated to salinity, which indicated temperature and salinity may play an important role in ciliate distribution. Indeed, ciliates showed significant positive correlation with Chl-a concentrations and inorganic nutrients (PO43-, NO2-, NO3- and NH4+). This may be due to the fact that many ciliates are consumers of phytoplankton in the microbial food web. The growth and proliferation of phytoplankton reduce and deplete the amounts of inorganic nutrients and at the same time enhance the growth of ciliates due to increased food availability.
Conclusion
The results of this study demonstrated that ciliate communities in the south coast of Sfax show significant spatial patterns, with high diversity in terms of Tintinnida composition, abundance, and biomass. The coupling of chemical and biological analysis may serve as a good means to monitor the marine ecosystem.
Acknowledgement
This study was conducted by Zohra BEN SALEM as a part of her Master research directed at the Biodiversity and Aquatic Ecosystems UR/11ES72 research unit at the University of Sfax, Tunisia.
Images and Tables
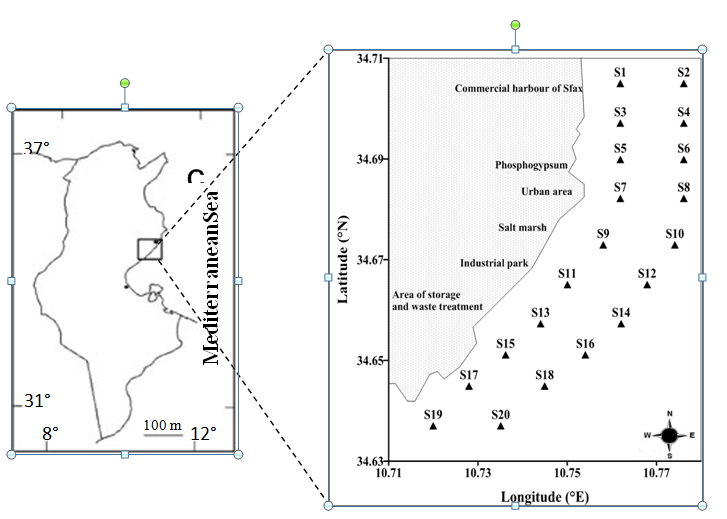
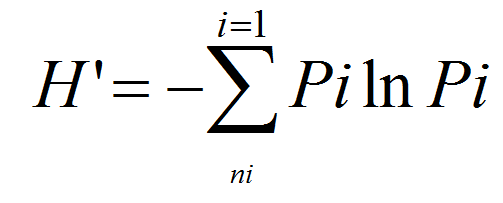
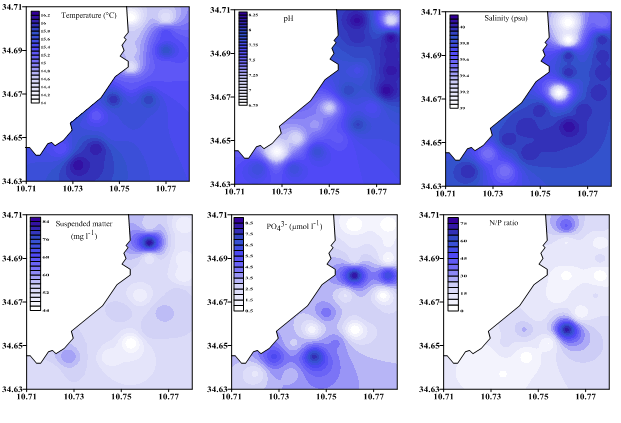
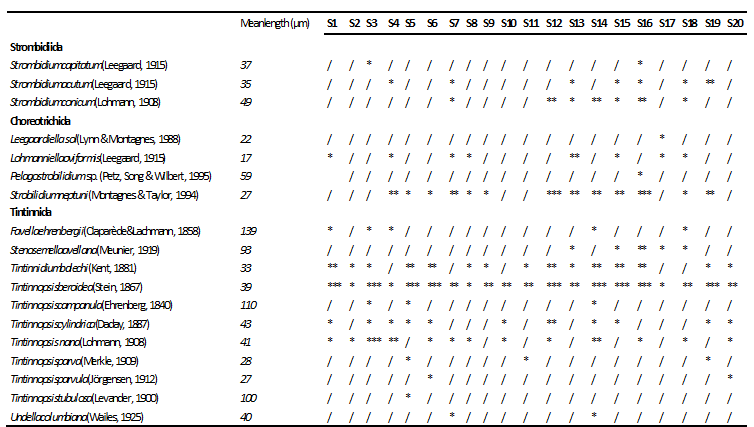
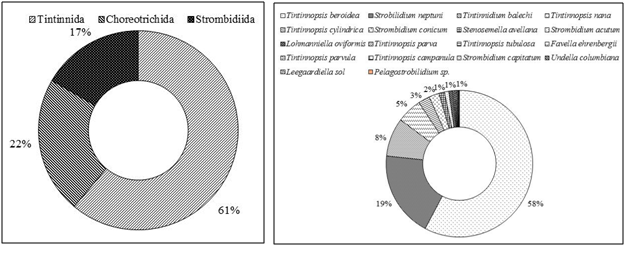
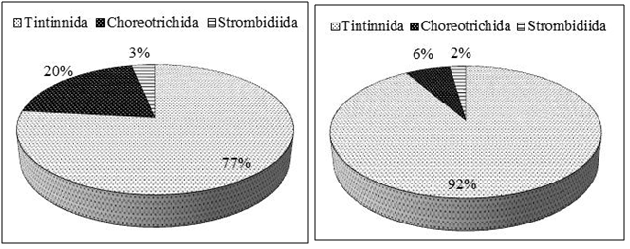
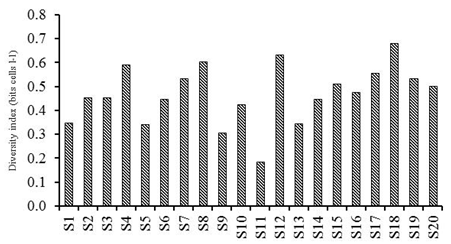
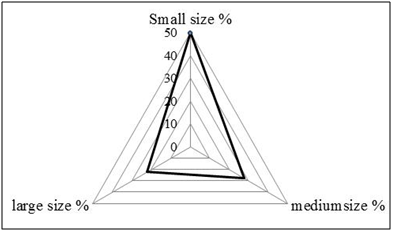
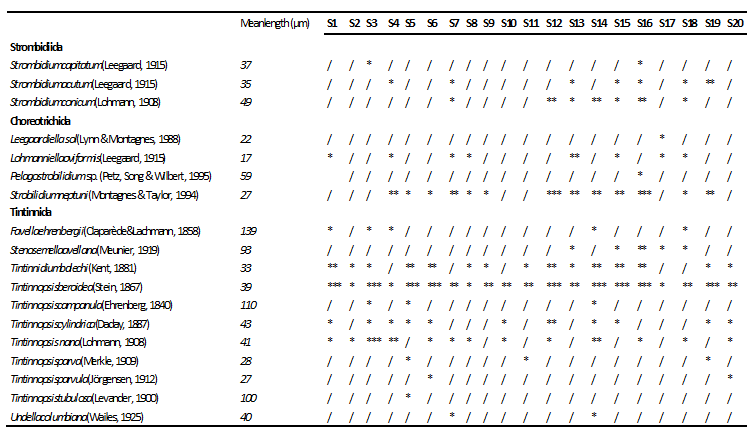
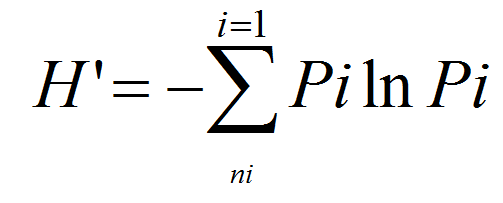
References
Azam F, Frenchel T, Field JG, Gray JS, Meyer-Reil LA, Thingstad F. The ecological role of water-column microbes in the sea. Mar Ecol Prog Ser. 1983;10:257?63.
Montagnes D, Allen J, Brown L, Bulit C, Davidson R, Fielding S, et al. Role of ciliates and other microzooplankton in the Irminger Sea (NW Atlantic Ocean). Mar EcolProg Ser;411:101?15. Available from:
View ArticleDolan JD, Marrac? C. Planktonic ciliate distribution relative to a deep chlorophyll maximum: Catalan Sea, N.W. Mediterranean, June 1993. Deco-Sea Fkearch I. 1995;42(11-12):1965?87.
Corliss JO. Biodiversity and biocomplexity of the protists and an overview of their significant roles in maintenance of our biosphere. Acta Protozool;41(3):199?220.
Stoecker DK, Capuzzo JM. Predation on protozoa: its importance to zooplankton. J Plankton Res;12(5):891?908.
Gong J, Song W, Warren A. Periphytic ciliate colonization: annual cycle and responses to environmental conditions. Aquat Microb Ecol;39(2):159?70.
Jiang X, Teng A, Xu W, Liu X. Distribution and pollution assessment of heavy metals in surface sediments in the Yellow Sea. Mar Pollut Bull;83(1):366?75.
Pratt JR, Crains JJ. Functional groups in the protozoa: roles in differing ecosystems. J Protozool. 1985;32:415?23.
Kchaou N, Elloumi J, Drira Z, Hamza A, Ayadi H, Bouain A, et al. Distribution of ciliates in relation to environmental factors along the coastline of the Gulf of Gabes, Tunisia. Estuar Coast Shelf Sci;83(4):414?24.
Wickham SA, Steinmair U, Kamennaya N. Ciliate distributions and forcing factors in the Amundsen and Bellingshausen Seas (Antarctic). Aquat Microb Ecol. 2011;62:215?30.
Zaghden H, Kallel M, Louati A, Elleuch B, Oudot J, Saliot A. Hydrocarbons in surface sediments from the Sfax coastal zone, (Tunisia) Mediterranean Sea. Mar Pollut Bull;50(11):1287?94.
Waycott M, Duarte CM, Carruthers TJ, Orth RJ, Dennison WC, Olyarnik S, et al., others. Accelerating loss of seagrasses across the globe threatens coastal ecosystems. ProcNatlAcad Sci;106(30):12377?81
Hamza-Chaffai A, Cosson RP, Amiard-Triquet C, El Abed A. Physico-chemical forms of storage of metals (Cd, Cu and Zn) and metallothionein-like proteins in gills and liver of marine fish from the Tunisian coast: ecotoxicological consequences. Camp Biochem Physiol. 1995;111(2):329?41.
Zaghden H, Kallel M, Elleuch B, Oudot J, Saliot A, Sayadi S. Evaluation of hydrocarbon pollution in marine sediments of Sfax coastal areas from the Gabes Gulf of Tunisia, Mediterranean Sea. Environ Earth Sci;72(4):1073?82
Serbaji MM, Azri C, Medhioub K. Anthropogenic contributions to heavy metal distributions in the surface and sub-surface sediments of the northern coast of Sfax, Tunisia. Int J Environ Res;6(3):613?26.
Louati A, Elleuch B, Kallel M, Saliot A, Dagaut J, Oudot J. Hydrocarbon contamination of coastal sediments from the Sfax area (Tunisia), Mediterranean Sea. Mar Pollut Bull;42(6):444?51.
Bourrelly P. Les Algues d?Eau Douce. Initiation ? la Syst?matique. Tome II . Les Algues bleues et rouges. Les Eugl?nins, Peridiniens et Cryptomonadines. 1985. 606 p.
UNESCO. Determination of Photosynthetic Pigments in Sea-water. Unesco, Paris; 1966.
Uterm?hl H. Zur Vervollkommnung der quantitativen Phytoplankton-Methodik. 1958. 38 p.
Balech E. Tintinnoinea del Mediterraneo. 1959. 88 p.
Kofoid CA, Campbell AS. A Conspectus of the Marine and Fresh-water Ciliata Belonging to the Suborder Tintinnoinea: With Descriptions of New Species Principally from the Agassiz Expedition to the Eastern Tropical Pacific 1904-1905. 1929. 1-403 p.
Kofoid CA, Campbell AS. The Tintinnoinea of the eastern tropical Pacific. Bull Mus Comp Zool Harv Coll. 1939;84:1?473.
Putt M, Stoecker DK. An experimentally determined carbon?: volume ratio for marine ?oligotrichous? ciliates from estuarine and coastal waters. Limnol Oceanogr. 1989;34:1097?103.
Shannon CE, Weaver G. The Mathematical Theory of Communication. University of Illinois Press. Urbana, Chicago; 1949.
Comin FA, Valiela I. On the Controls of Phytoplankton Abundance and Production in Coastal Lagoons. J Coast Res. 1993;9:895?906.
Ben Ltaief T, Drira Z, Hannachi I, Bel Hassen M, Hamza A, Pagano M, et al. What are the factors leading to the success of small planktonic copepods in the Gulf of Gabes, Tunisia? J Mar BiolAssoc U K;95(04):747?61.
Drira Z, Bel Hassen M, Ayadi H, Aleya L. What factors drive copepod community distribution in the Gulf of Gabes, Eastern Mediterranean Sea? Environ Sci Pollut Res;21(4):2918?34.
Thingstad TF, Zweifel UL, Rassoulzadegan L. P limitation of heterotrophic bacteria and phytoplankton in the northwest Mediterranean. Limnol Oceanogr. 1998;43:88?94.
Bel Hassen M, Drira Z, Hamza A, Ayadi H, Akrout F, Issaoui H. Summer phytoplankton pigments and community composition related to water mass properties in the Gulf of Gabes. Estuar Coast Shelf Sci;77(4):645?56.
Drira Z, Belhassen M, Ayadi H, Hamza A, Zarrad R, Boua?n A, et al. Copepod community structure related to environmental factors from a summer cruise in the Gulf of Gab?s (Tunisia, eastern Mediterranean Sea). J Mar Biol Assoc U K;90(01):145.
Vollenweider RA, Marchetti R, Viviani R. Marine Coastal Eutrophication: The Response of Marine Transitional Systems to Human Impact?: Problems and Perspectives for Restoration?: Proceedings. Amsterdam?, New York; 1993.
Dhib A, Brahim MB, Ziadi B, Akrout F, Turki S, Aleya L. Factors driving the seasonal distribution of planktonic and epiphytic ciliates in a eutrophicated Mediterranean Lagoon. Mar Pollut Bull;74(1):383?95.
Hannachi I, Drira Z, Belhassen M, Hamza A, Ayadi H, Bouain A, et al. Abundance and biomass of the ciliate community during a spring cruise in the Gulf of Gabes (Eastern Mediterranean Sea, Tunisia). Acta Protozool;47(4):293.
P?rez MT, Dolan JR, Vidussi F, Fukai E. Diel vertical distribution of planktonic ciliates within the surface layer of the NW Mediterranean (May 1995). Deep Sea Res Part Oceanogr Res Pap;47(3):479?503.
Abboud-Abi Saab M. Tintinnids of the Lebanese Coastal Waters (Eastern Mediterranean). Lebanon; 2008. 192 p.
Yang EJ, Ha HK, Kang S-H. Microzooplankton community structure and grazing impact on major phytoplankton in the Chukchi sea and the western Canada basin, Arctic ocean. Deep Sea Res Part II Top Stud Oceanogr;120:91?102.
Dolan JR. Tintinnid ciliate diversity in the Mediterranean Sea: longitudinal patterns related to water column structure in late spring?early summer. Aquat Microb Ecol. 2000;22:69?78.
Dolan JR, Claustre H, Carlotti F, Plounevez S, Moutin T. Microzooplankton diversity: relationships of tintinnid ciliates with resources, competitors and predators from the Atlantic Coast of Morocco to the Eastern Mediterranean. Deep-Sea Res I. 2002;49:1217?32.
Hannachi I, Drira Z, Bel Hassen M, Hamza A, Ayadi H, Aleya L. Species composition and spatial distribution of abundances and biomass of phytoplankton and ciliates during summer stratification in the Gulf of Hammamet (Tunisia). J Mar BiolAssoc U K;91(07):1429?42.
Dolan JR, Jacquet S, Torr?ton JP. Comparing taxonomic and morphological biodiversity of tintinnids (planktonic ciliates) of New Caledonia. Limnol Oceanogr. 2006;51:950?8.
Modigh M, Castaldo S. Variability and persistence in tintinnid assemblages at a Mediterranean coastal site. Aquat Microb Ecol. 2002;28:299?311.
P?rez MT, Dolan JR, Fukai E. Planktonic oligotrich ciliates in the NW Mediterranean: growth rates and consumption by copepods. Mar Ecol Prog Ser. 1997;155:89?101.
Telesh IV. Plankton of the Baltic estuarine ecosystems with emphasis on Neva Estuary: a review of present knowledge and research perspectives. Mar Pollut Bull;49(3):206?19.
Ismael AA, Dorgham MM. Ecological indices as a tool for assessing pollution in El-Dekhaila Harbour (Alexandria, Egypt). Oceanologia;45(1):121?31.
Wilhm JL. River ecology. In: Whitton BA, editor. Biological indicators of pollution. Blackwell Publishing. Oxford; 1975. p. 375?402.