Abbasi S Email: sabbasifood@modares.ac.ir
Designing a microwave-assisted low pressure cold plasma (LPCP) generator: A case study on Salmonella decontamination
Corresponding Author
Affiliation
Harouni A, Abbasi* S
Food Colloids and Rheology Laboratory, Department of Food Science & Technology, Faculty of Agriculture, Tarbiat Modares University, P. O. Box 14115-336, Tehran, Iran.
Article Reviewed By:
Dongping Liu(Dongping.liu@dlnu.edu.cn)
Nicolas Jidenko(nicolas.jidenko@u-psud.fr)
Jue Zhang(zhangjue@pku.edu.cn)
Citation
Soleiman Abbasi, Designing a microwave-assisted low pressure cold plasma (LPCP) generator: A case study on Salmonella decontamination(2017)SDRP Journal of Food Science & Technology 2(2)
Abstract
The main objective of the present study was to develop a microwave-assisted low pressure cold plasma (LPCP) generator to be used for decontamination purposes. After the successful designing of the generator and production of cold plasma, optimum operating conditions were experimentally determined. Then, its efficacy on decontamination of egg shell and aluminum foil (inoculated by Salmonella enterica subspp. enterica PTCC: 1709) under various LPCP exposure and storage times was investigated. LPCP exposure for 5 min diminished the bacterial counts by 1.92 and 3.2 logs on the egg shell and aluminum foil, respectively. Moreover, the LPCP exposure up to 10 min showed no significant effect (p>0.05) on various qualitative characteristics, while prolonged LPCP treatment accelerated pH increment in albumen. The achievements of the present study represented the potential of LPCP generator for the partial decontamination of various food and packaging items as an appropriate alternative to the thermal or chemical methods.
Keywords: Cold Plasma, Microwave, Salmonella, Minimal Processing.
Introduction
Salmonella spp. are pathogenic foodborne bacteria which impose some social, safety and economy concerns worldwide, especially in developing nations (Liu et al., 2013). It is reported that almost 1.5 million of the infections, thousands of hospitalizations and hundreds of deaths caused annually by Salmonella in the USA. Such foodborne outbreak has an annual cost of around $7 billion and Salmonella acts an imperative role as the second common foodborne pathogenic microorganism (Bermudez-aguirre and Corradini, 2012). The main Salmonellosis symptoms are nausea, vomiting, abdominal pain, diarrhea and fever (Mukhopadhyay and Rmaswamy, 2012). Salmonella is able to grow and survive both in wide range of food items and environmental circumstances like low moisture and thermal processing (Bermudez-aguirre and Corradini, 2012). For example poultry, raw meat, milk and egg products are the desired foods to carry these bacteria which do not usually influence the organoleptic properties of the host (Mukhopadhyay and Rmaswamy, 2012).
It is reported that surface contamination of eggs by Salmonella is a potential risk to consumers and egg handlers. As a result, decontamination of eggs is indispensable operation which currently performed by harmful chemicals or fumigation. These operations may cause a number of health-threating concerns along with the damage to egg cuticle which can reduce its shelf life (Davies and Breslin, 2003). In addition, some functional characteristics of egg and egg products can be impaired by heat treatments; hence pasteurization treatment should be done in a low temperature long time regime (3.5 min at 56.6˚C for albumen, 3.5 min at 60˚C for whole egg and 3.5 min at 61.1˚C for egg yolk) to inhibit protein coagulation. Nevertheless, it should be considered that consumers generally prefer minimally processed and preservative-free products. Consequently, introducing an alternative non-thermal decontamination technique which guarantees the product quality is crucial (De Souza and Fernandez, 2011). One of these techniques is cold plasma, a novel and efficient technique, which reduces the pathogenic bacteria of eggshell and prevents any potential risks for humans (Pasquali et al., 2010).
Plasma, the fourth state of the matter which is known as a neutral ionized gas, is produced by permanently interacted particles including photons, atoms, electrons, ions, excited and/or non-excited molecules and free radicals. In the classification of plasma-fabricating particles, photons and electrons considered as light-type species whilst others considered as heavy-type species. Accordingly, plasma defined as energetic transfer to a gas as a result of neutralization or ionization of heavy-type species (Moreau et al., 2008). Both light and heavy species can interact with a wide range of microorganism cells (bacteria, spores and viruses) and efficiently inactive those (Ulbin-Figewicz et al., 2015). In term of temperature, there are two types of plasma namely hot and cold plasmas. Plasma with temperature of near room temperature even in atmospheric or reduced pressure is known as "cold plasma" (Niemira, 2012a). Dielectric barrier discharge (DBD), jet plasma and Corona discharge are the common atmospheric pressure cold plasmas that have been used in food systems. For example, DBD is already used in decontamination of tomatoes (Pankaj et al., 2013), pericarp of fruits (Perni et al., 2008) and meat (Rød et al., 2012). Corona discharge is also utilized for decontamination of milk (Gurol et al., 2012) and active packaging of apple (Fernandez-Gutierrez et al., 2010). Regarding jet plasma, it has shown significant effects on decontamination of almond nut (Niemira, 2012b). Cold plasma also used to decontaminate various bacteria on artificial packaging-related surfaces such as glass, paper (Moisan et al., 2002), nitrocellulose (Purevdorj et al., 2002) and polypropylene (Lee et al., 2006).
Exploiting low pressure cold plasma (LPCP) is lower than atmospheric cold plasma due mainly to the high cost. The LPCP is mainly generated by microwaves. In this system, the plasma reactor chamber, containing certain gases under low pressure, is placed inside a microwave emitting chamber. Then, the produced energy conveys to the gas by an antenna and the gas turn into plasma. Oxygen, N2O and argon are the most commonly used gases to generate plasma. So far, the LPCP has shown reasonable capability to destroy the sensitive vegetative varieties of E. coli as well as the resistant spores of Bacillus stearothermophilus in several food products (Morear et al., 2000).
Therefore, the main objectives of the present study were to design and construct a prototype microwave-assisted LPCP in order to surface decontamination of deliberately contaminated egg shells (as a food product) and aluminum foil (as a food packaging material) by Salmonella enterica sub spp. enterica under different plasma exposure time and storage time. Some quality attributes and shelf life of eggs are also evaluated.
Materials & Methods
Chemicals and microorganisms
Nutrient broth (NB), xylose lysine deoxycholate (XLD) agar, and plate count agar (PCA) were obtained from Merck Co (Darmstadt, Germany). Fresh eggs acquired from the poultry farm of Tarbiat Modares University (TMU). Ethanol and NaCl were obtained from Kimiadaru (Tehran, Iran) and aluminum foils were purchased from Iran-alumina (Tehran, Iran). Salmonella enterica subspp. enterica (PTCC: 1709) was purchased from the Culture Collection Center of The Iranian Research Organization for Science and Technology (IROST, Tehran, Iran). Other chemicals used in this study were of analytical grade.
Designing LPCP generator
Fig. 1 illustrates the cold plasma generator device which was designed by ourselves at TMU. This system mainly consists of four essential parts: 1) the gas pressure and flow monitoring, 2) the plasma source (domestic microwave oven), 3) the afterglow chamber where the objects to be sterilized are placed, and 4) a vacuum pumping system.
The flow of inlet gas is controlled by a needle valve (Model NV10-01, Yar-Nikan-Saleh Engineering Company, Tehran, Iran) and monitored by a flow-meter (Model DK800-04, Arshia Engineering Company, Tehran, Iran). The inner pressure of the plasma reactor is monitored by a vacuum meter (Model PM-9107, Lutron, Taiwan). The domestic microwave oven (AEG Micromat, 1200W, 2.45 GHz, Nürnberg, Germany) is used as energy source for plasma fabrication inside the glow discharge tube (Pyrex, 45 mm diameter and 210 mm length). The afterglow chamber (Pyrex, 75 mm diameter and 210 mm length) is connected to the glow discharge tube, covered by aluminum foils, and located outside of the microwave oven chamber. The samples (e.g. egg, aluminum foil, etc.) which need to be sterilized are located inside afterglow chamber using different holders (Fig. 2). It needs to be noted that one end of afterglow chamber was connected to a vacuum pump (Edward RV3, England) and the other end was connected to the glow discharge tube. As a result, the required pressure (5 mbar) inside the afterglow chamber and glow discharge tube was adjusted by vacuum pump, as outlet, as well as the flow rate of inletting gases.
In order to verify the effectiveness of the designed cold plasma generator for superficial decontamination of egg shell, as a food sample, and aluminum foil, as a packaging material, a target pathogen has been intentionally inoculated on their surfaces according to subsection 2.3.2. Then, they were placed inside the afterglow chamber and decontaminated by LPCP treatment at different time periods namely: 0, 1, 2, 3, 4, and 5 min.
Microbiological procedures
Bacterial culture and inoculum preparation
To do so, the strain was cultured in NB at 37 ͦC for 24 h with a slight agitation (100 rpm). Then, an aliquot of the grown culture (10g kg-1 inoculum) was subsequently transferred into 200 ml of NB and incubated at 37 ͦC for 24 h with slight agitation. The grown cultures were transferred into five sterile falcon tubes, and cells were harvested by centrifugation (1950×g, 10 min, at 4 ͦC). Finally, the media were discarded and the harvested cells re-suspended in 10 ml of NaCl solution (9 g kg-1) and kept in refrigerator until use. The concentration of microorganism in suspension was 1.5×109 CFU/ml (colony forming unit/ml).
Inoculation of egg shell and aluminum foil
At first, eggs were washed with distilled deionized water (at room temperature), followed by a sanitization by dipping in ethanol (70%, v/v) for 30 min according to Ragni et al., (2010). Then, the sanitized eggs were transferred to a container under a sterile laminar hood and aseptically dried at room temperature for about 40 min before inoculation. They were inoculated by 100 μl of the prepared microbial suspension in the form of drops located on egg shell by a sampler pipette and then air dried for approximately 1 h before being exposed to the cold plasma treatment. This procedure steadily provided microbial concentration on the shell at levels ranging from 6.5 to 7 log CFU. The aluminum foils (2 × 3 cm pieces) were also sterilized (121 ºC for 30 min) then inoculated by 100 μl of the prepared microbial suspension in the form of drops located on each foil piece by sampler pipette; and then air dried for approximately 1 h before being exposed to the plasma source. The average microbial count on the surface of aluminum foils was 7.5–8.5 log CFU.
Viable cell counts
After the plasma treatments, eggs were transferred into a sterile polyethylene bag and 20 ml of sterile saline solution was added. They were then hand-rubbed through the bag for 5 min to detach the bacteria (De Reu et al., 2006) and 1 ml aliquots were used to prepare decimal serial dilutions. For enumeration of viable cells on the surface of foils, foils were transferred into a test tube containing 10 ml sterile saline solution under aseptic conditions. The test tube was then shaked for 5 min to detach the bacteria and a 1 ml aliquot was used to prepare decimal serial dilutions. Viable cell counting was done by Miles-Misra technique (surface drop method) as described by Harrigan (1998), in which 20 μl of the appropriate dilutions was poured into the non-selective PCA and selective XLD-agar plates which followed by an incubation at 37 ͦC for 24–48 h.
Quality attributes of eggs
LPCP treated eggs characterized after 0, 7, 15 and 30 days storage at room temperature. The weight loss (as an indirect method to evaluate the shell membrane integrity), albumin pH, albumin height, Haugh unit (HU) index, yolk color, shell thickness, shell breaking strength was measured. The albumin pH was measured by a pH meter (Metrohm 744, Herisau, Switzerland) on thick portion of albumin. The shell thickness of eggs was measured by an ultrasonic thickness gauge (Karl Deutsch Echometer 1061, Karl Deutsch Co. Ltd., Wuppertal, Germany). The shell strength was measured by a Digital Egg Shell Force Gauge (model-II) (FGX-5R, Robotmation, Tokyo, Japan). The albumin height, HU index, and yolk color was measured by Egg Multi Tester (EMT-5200, Robotmation Co. Ltd., Tokyo, Japan). The HU index is calculated by the following equation:
HU = 100 × Log (h-1.7w0.37+7.6)
Where h is the observed height of the albumen (mm) and w is the weight of egg (g).
Statistical analysis
The data obtained from different treatments were compared using analysis of variance (ANOVA) by SPSS software (Version 18). Significant differences, on the basis of p-value, (p<0.05) were performed by least significant difference (LSD). All measurements were carried out in triplicate.
Results
Optimization of cold plasma generation
The temperature measurement in three points of afterglow chamber showed that irradiation caused an abrupt increase followed by a slow increase in temperature at all points (Fig 3). According to our findings, the highest temperature (55.2°C) was observed after 15 min of plasma exposure at the midpoint of the afterglow chamber (exactly at its connection point to the discharge tube Fig 3a). However, the temperature of the eggs which were positioned at that point was reached 48.1°C after 15 min plasma treatment. It needs to be noted that the LPCP treatment period in this study was 5 min at the maximum. So, the temperature of the treated eggs even was far less than 48.1°C.
Fig. 4 also displays the influence of different pressure (5, 10, 15 and 20 mbar) levels on the integrity and intensity of generated plasma light. It is clear that once the pressure level inside the plasma chamber was fixed at 5 mbar, in that state, brighter and more uniform plasma light was observed in the glow discharge chamber. It is reported that the brightness and uniformity of plasma light is an indication of the maximum excitation of the molecules (Morear et al., 2000). It should be noted that 5 mbar pressure was reached when the needle valve was completely closed where there was no gas flow. Therefore, it can be concluded that despite the brightness and uniformity of plasma light at 5 mbar pressure it cannot be an ideal pressure for plasma treatments by different gases in the afterglow chamber. In contrary, when the gas flow rate was adjusted about 16 Nl/h the absolute pressure extended to 10 mbar. In this state, the plasma generator was in the excellent operation situation since both the vacuum was at the maximum level, and the inlet gas was sufficient enough to form plasma.
After optimization of gas flow rate and pressure level, in the next stage the influence of gas type (argon, nitrogen and air) on the visual characteristics of plasma light was investigated (Fig. 5). As it can be seen, the argon gas plasma was much more intense and uniform than the other two, which reflects a greater inclination of argon to generate plasma. Accordingly, argon with a flow rate of 16 Nl/h under 10 mbar absolute pressure were chosen as the optimum plasma formation conditions for the microbial decontamination of egg and packing foil as well as its effects on the qualitative parameters of plasma treated eggs.
Effect of LPCP on the survival of Salmonella
Fig. 6 represents the influence of different LPCP exposure time on the viability of Salmonella on the surface of eggs and aluminum foil on selective (XLD-agar) and nutritive (PCA) media. Regarding the eggs, the LPCP treatment time caused a significant (p<0.01) decline in the number of the inoculated Salmonella. The initial number of the inoculated bacteria also affected the microbial load of egg surface in a decreasing pattern (p <0.05). As can be seen, after 300 s plasma treatment, 1.75 and 1.95 log reduction were observed in PCA and XLD media, respectively. The average number of bacteria on the PCA was slightly higher than XLD. In line with our findings, Ragni and co-workers (2010) also concluded that in most cases, the number of survivals on selective medium was lower than non-selective or nutritive media. There is not any comprehensive report regarding the impacts of cold plasma on reduction of the microbial load but for instance, Davies and Breslin (2003) using the ionized gas (plasma) noticed no substantial reduction on the number of inoculated bacteria. In contrary, Ragni and co-workers (2010) reported 5.2 and 5.4 log reductions on microbial loads in relatively low and high moisture conditions using RBD plasma, respectively.
The decontamination results of LPCP on aluminum foils was almost similar to egg shell (p <0.01), nevertheless the effect of the initial number of inoculated bacteria was not as good as egg shell. All in all, foil exposure (5 min) to LPCP led to 3.2 and 3.1 log reduction in the XLD and PCA media, respectively. It seems that the high efficacy of microwave-assisted LPCP on aluminum foil (3.2 log) compared to egg shell (1.9 log) is associated with their topographical differences. In other words, the egg shells are usually uneven due to the existence of many bumps and pores and these could possibly play a protective role for the inoculated microorganisms. In a recent study, Yun et al. (2010) also reported a 3 log reduction on aluminum foil when treated by cold plasma for 120 s.
Influence of LPCP treatment on some quality parameters of eggs
Table 1 indicates the influence of LPCP exposure (0, 1, 5, 10 and 15 min) and storage time (0, 15 and 30 days) on quality attributes of the treated eggs. Based on our findings, the albumen height value significantly (p<0.05) decreased as the storage time increased whilst the LPCP exposure time was not effective (p<0.05). The interaction of LPCP exposure time and storage time was not also significant (p<0.05). In addition, the most of these changes happened in the first two weeks of storage. These results were in consistent with the previous reports (Scott and Silversides, 2000; Silversides and Scott, 2001). Jones and Musgrove (2005) have also reported that the effect of storage time was significant during the first 10 days so that the albumen height dropped from 7.05 to 4.75 mm.
The singular effects of LPCP exposure time and storage time on HU index was significant (p<0.05) however, their interaction was not (p>0.05). It needs to be noted that the reducing effect of LPCP exposure on the HU index only happened after15 min. No report was found concerning the impacts of cold plasma on HU index. Nevertheless, Jones and Musgrove (2005) and Jin et al. (2011) have also observed a comparable decline during storage.
The LPCP exposure had no significant effect on yolk color while by increasing the storage time the yolk color number increased, in the other words, the yolk color decreased. Jin and co-workers (2011) in their study on effect of storage temprature and time noticed a significant decrease within 10 days of storage.
Regarding the thickness of egg shells it was noticed that LPCP exposure was not effective on itself but it became thinner after 15 days. However, the thickness value was astonishingly close to the initial thickness (at the beginning of LPCP treatment) after 30 days of storage. Moreover, their interactive effect was not also significant (p>0.05).
Similar to the other parameters, egg weight loss was not observe by LPCP exposure times (p>0.05), but an increasing pattern (from 0.08% to 10.39%) observed by prolonging storage time. Their interaction was not also significant (p>0.05). Jones and Musgrove (2005) reported analogous trend in increasing weight loss during storage time. The influence of LPCP exposure, storage times and their interactions did not show any significant effect on shell breaking strength.
Fig. 7 demonstrates the effect of LPCP exposure and storage times on egg albumen pH. As it can be seen, not only LPCP exposure but also storage time intervals and their interaction had significant effects (p<0.05) on the pH value of albumen. It is interesting that the increase in the pH of LPCP treated eggs wasn’t significant immediately after treatment but during storage it significantly (p<0.05) changed. However, the longer the exposure time the worse the effects on pH as 15 min LPCP treatment was much worse than 10, 5, or 1 min. In contrary to our results, it was stated20 that 90 min treatment of eggs by RBD plasma hasn’t significant effects on albumen pH. These differences could be attributed to the fundamental differences on the nature of plasmas which could be produced by these two plasma production techniques. With regard to albumen pH, Jin and co-workers (2011) reported an increase by increasing the temperature and storage time.
Conclusion
The results of the current study have shown that microwave-assisted LPCP generator which was designed could fabricate afterglow cold plasma under the optimized processing conditions for sterilization processes. Based on our achievements, it can satisfactorily decontaminate Salmonella Spp. from the surface of eggs as a food product and aluminum foils as packaging materials. There was 1.92 and 3.2 log reductions in the number of deliberately inoculated bacteria after 5 min LPCP treatment on the surface of egg shell and aluminum foil, respectively. Furthermore, no considerable negative quality aspects were found up to 10 min. All in all, it can be concluded that the designed plasma generator was able to partially decontaminate food products and packaging materials in low temperature and short times. It seems by doing further improvements and optimizations, this generator can be scaled-up at very low price for industrial applications too.
Images and Tables
Treatment |
Quality parameter |
||||||
Storage time (day) |
LPCP exposure time (min) |
albumen pH |
shell strength (Pa) |
shell thickness (mm) |
yolk color number |
HU index |
albumen height (mm) |
0 |
0 |
7.82h |
2.68a,A |
0.31a,A |
4.33a,B |
80.60a,A |
6.70a,A |
1 |
8.09gh |
3.05a,A |
0.31a,A |
4.00a,B |
85.93a,A |
7.20a,A |
|
5 |
7.77h |
3.77a,A |
0.32a,A |
4.00a,B |
71.73a,A |
5.40a,A |
|
10 |
7.95gh |
3.38a,A |
0.34a,A |
4.00a,B |
82.10a,A |
7.14a,A |
|
15 |
7.99gh |
2.77a,A |
0.32a,A |
4.67a,B |
66.13b,A |
4.97a,A |
|
15 |
0 |
8.25gh |
3.29a,A |
0.28a,B |
3.50a,B |
41.80a,B |
3.05a,B |
1 |
8.65efg |
2.00a,A |
0.29a,B |
4.67a,B |
49.00a,B |
3.00a,B |
|
5 |
8.76def |
1.93a,A |
0.29a,B |
4.67a,B |
40.50a,B |
2.50a,B |
|
10 |
8.56fg |
2.52a,A |
0.28a,B |
4.00a,B |
45.83a,B |
2.90a,B |
|
15 |
8.73bc |
3.22a,A |
0.29a,B |
4.00a,B |
17.60b,B |
2.05a,B |
|
30 |
0 |
8.69fgh |
2.41a,A |
0.32a,AB |
5.00a,A |
50.60a,C |
3.10a,B |
1 |
8.69cde |
2.67a,A |
0.32a,AB |
5.00a,A |
40.10a,C |
2.20a,B |
|
5 |
8.63cd |
2.39a,A |
0.31a,AB |
6.00a,A |
59.30a,C |
3.50a,B |
|
10 |
9.42a |
3.47a,A |
0.31a,AB |
4.50a,A |
42.80a,C |
2.25a,B |
|
15 |
9.29ab |
3.22a,A |
0.33a,AB |
4.50a,A |
40.25b,C |
2.40a,B |
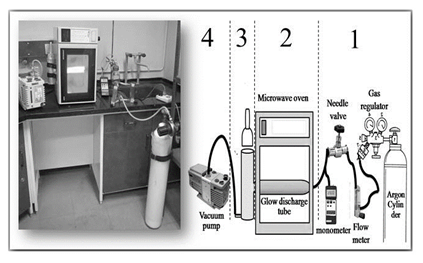
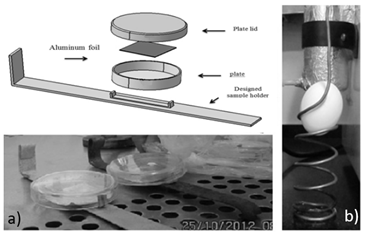
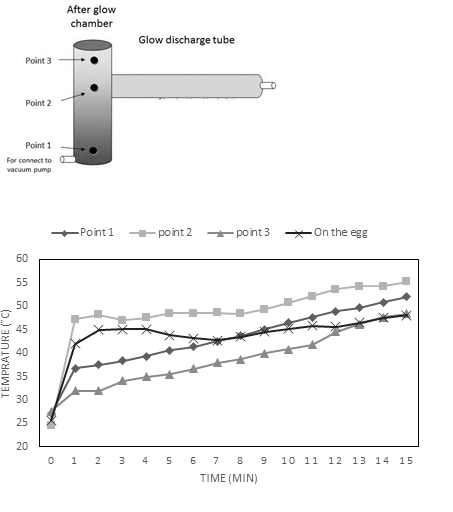
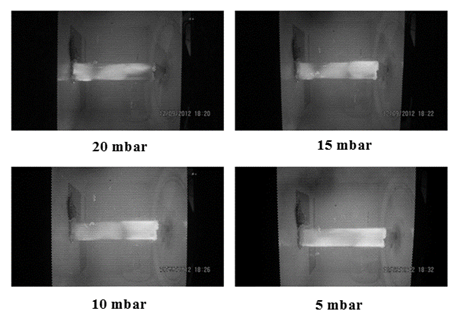
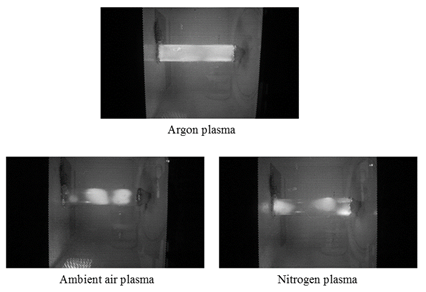
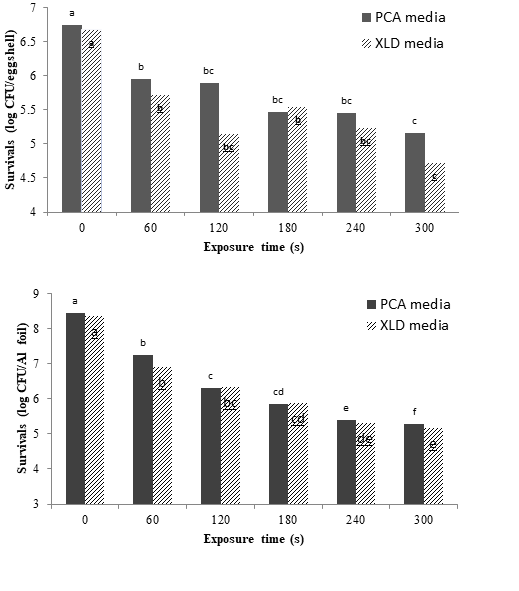
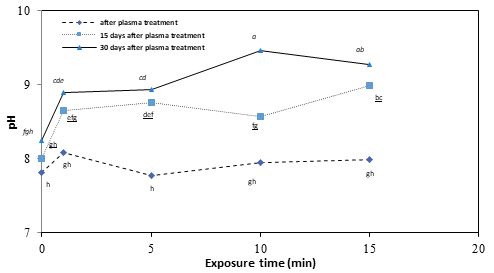
References
Bermudez-aguirre D and Corradini MG, (2012). Inactivation kinetics of Salmonella spp. under thermal and emerging treatments: A review. Food Res Int 45, 700-712.
View ArticleDavies RH and Breslin M, (2003). Investigations into possible alternative decontamination methods for Salmonella enteritidis on the surface of table eggs. J Vet Med B 50, 38-41.
View ArticleDe Reu K, Grijspeerdt K, Messens W, Heyndrickx M, Uyttendaele M, Debevere J and Herman L, (2006). Eggshell factors influencing eggshell penetration and whole egg contamination by different bacteria, including Salmonella enteritidis. Int Journal Food Microbiol 112, 253-260. PMid:16822571
View Article PubMed/NCBIDe Souza PM and Fernandez A, (2011). Effects of UV-C on physicochemical quality attributes and Salmonella enteritidis inactivation in liquid egg products. Food Control 22, 1385-1392.
View ArticleFernandez-Gutierrez SA, Pedrow PD, Pitts MJ and Powers J, (2010). Cold atmospheric-pressure plasmas applied to active packaging of apples. Plasma Sci, IEEE Transactions on 38, 957-965.
View ArticleGurol C, Ekincl F, Aslan N and Korachi M, (2012). Low temperature plasma for decontamination of E. coli in milk. Int J Food Microbiol 157, 1-5. PMid:22622128
View Article PubMed/NCBIHarrigan WF (1998). Laboratory Methods in Food Microbiology, Acad. Press.
Jin Y, Lee K, Lee W and Han Y, (2011). Effects of storage temperature and time on the quality of eggs from laying hens at peak production. Asian-Aust J Anim Sci 24, 279-284.
View ArticleJones D & Musgrove M, (2005). Effects of extended storage on egg quality factors. Poult Sci 84, 1774-1777 PMid:16463976
View Article PubMed/NCBILee K, Paek KH, Ju W and Lee Y, (2006). Sterilization of bacteria, yeast, and bacterial endospores by atmospheric-pressure cold plasma using helium and oxygen. J Microbiol-Seoul 44, 269-276.
Liu C, Hofstra N and Franz E (2013). Impacts of climate change on the microbial safety of pre-harvest leafy green vegetables as indicated by Escherichia coli O157 and Salmonella spp. Int J Food Microbiol 163, 119-128. PMid:23558195
View Article PubMed/NCBIMoisan M, Barbeau J, Crevier MC, Pelletier J, Philip N and Saoudi B, (2002). Plasma sterilization. Methods and mechanisms. Pure Appl Chem 74, 349-358.
View ArticleMorear M, Moisan M, Tabrizian M, Barbeau J, Pelletier J, Ricard A and Yahia LH, (2000). Using the flowing afterglow of a plasma to inactivate Bacillus subtilis spores: Influence of the operating conditions. J Appl Phys 88, 1166-1174.
View ArticleMoreau M, Orange N and Feuilloley MGJ, (2008). Non-thermal plasma technologies: New tools for bio-decontamination. Biotech Adv 26, 610-617. PMid:18775485
View Article PubMed/NCBIMukhopadhyay S and Rmaswamy R, (2012). Application of emerging technologies to control Salmonella in foods: A review. Food Res Int 45, 666-677.
View ArticleNiemira BA, (2012a). Cold plasma decontamination of foods. Annu Rev Food Sci Technol 3, 125-142. PMid:22149075
View Article PubMed/NCBINiemira BA, (2012b). Cold plasma reduction of Salmonella and Escherichia coli O157:H7 on almonds using ambient pressure gases. J Food Sci 77, 171-175. PMid:22384964
View Article PubMed/NCBIPankaj S, Misra N and Cullen P, (2013). Kinetics of tomato peroxidase inactivation by atmospheric pressure cold plasma based on dielectric barrier discharge. Innovative Food Sci & Emerging Technol 19, 153-157.
View ArticlePasquali F, Fabbri A, Cevoli C, Manreda G and Franchini A, (2010). Hot air treatment for surface decontamination of table eggs. Food Control 21, 431-435.
View ArticlePerni S, Shama G and Kong MG, (2008). Cold atmospheric plasma disinfection of cut fruit surfaces contaminated with migrating microorganisms. J Food Prot 71, 1619-1625.
View ArticlePurevdorj D, Igura N, Hayakawa I and Ariyada O, (2002). Inactivation of Escherichia coli by microwave induced low temperature argon plasma treatments. J Food Eng 53, 341-346. 00174-1
View ArticleRagni L, Berardinelli A, Vannini L, Montanari C, Sirri F, Guerzoni ME and Guarnieri A, (2010). Non-thermal atmospheric gas plasma device for surface decontamination of shell eggs. J Food Eng 100, 125-132.
View ArticleR?d SK, Hansen F, Leipold F and Kn?chel S, (2012). Cold atmospheric pressure plasma treatment of ready-to-eat meat: Inactivation of Listeria innocua and changes in product quality. Food Microbiol 30, 233-238. PMid:22265306
View Article PubMed/NCBIScott T and Silversides F, (2000). The effect of storage and strain of hen on egg quality. Poult Sci 79, 1725-1729 PMid:11194033
View Article PubMed/NCBISilversides F and Scott T, (2001). Effect of storage and layer age on quality of eggs from two lines of hens. Poult Sci 80, 1240-1245.
View ArticleUlbin-Figewicz N, Jarmoluk A and Marycz K, (2015). Antimicrobial activity of low-pressure plasma treatment against selected foodborne bacteria and meat microbiota. Ann Microbiol 65, 1537-1546. PMid:26273240
View Article PubMed/NCBIYun H, Kim B, Jung S, Kruk ZA, Kim DB, Choe W and Jo C, (2010). Inactivation of Listeria monocytogenes inoculated on disposable plastic tray, aluminum foil, and paper cup by atmospheric pressure plasma. Food Control 21, 1182-1186.
View Article