Dmitri O Levitsky,aPaulina Goldshlag,bVM Dembitsky
Profiling of bioactive lipids of the wild edible land snails of the genus Helix
Co-Authors
Citation
valery dembitsky, Profiling of bioactive lipids of the wild edible land snails of the genus Helix (2016)SDRP Journal of Food Science & Technology 1(1)
Abstract
Pulmonate gastropod mollusks of the genus Helix are being used by human as food more than 10,000 years and they are quite important in the diet of many European countries. We investigated lipid composition of wild land snails sampled in France, Germany, Luxemburg, Norway, Switzerland, Sweden, and East Mediterranean inhabitants. Plasmalogens, glyceryl ethers, and diacyl phospholipid forms as well as their fatty aldehydes, alkyl ether glycerides, and fatty acid derivatives were studied. PE of snails, containing aldehydes C16 (variations from 17 to 40%), C18 (11-36%), C9-18:1 (22-31%), C11-20:1 (1-3%), and several minor aldehydes, were detected. The major saturated 1-O-alkyl glycerol ethers were C16:0 and C18:0. Eicosatetraenoic (ETA, 34%), ?-linolenic acid (ALA, 14%), and eicosapentaenoic acid (EPA, 7%) in PE (all forms) were dominating fatty acids. ETA (49%), EPA (13%), ALA (7%), and docosahexaenoic (DHA, 6%) were major fatty acids in PS (all forms). In PC (all forms), major fatty acids were found oleic (20%), palmitic (15%), ETA (14%), and linoleic (10%). In neutral plasmalogens, the predominant fatty acids were palmitic acid (29%), oleic acid (13%), ALA (8%), and ETA (7.8%). Predominant fatty acids in neutral plasmalogens were found to be 16:0 (29%), C18:0 (13%), ALA (8%), and ETA (7.8%). Distribution of plasmalogens, alkyl glyceryl ethers, and their fatty aldehydes and fatty alcohols in gastropod species and other mollusks is also discussed.
Introduction
ABBREVIATIONS
PAHs (polycyclic aromatic hydrocarbons), NAPLs (Non-aqueous phase liquids), HOCs (hydrophobic organic compounds), LNAPL (light non-aqueous phase liquid), DNAPL (dense non-aqueous phase liquid), IFT (interfacial tension), PCBs (polychlorinated biphenyls), EP (extreme pressure), ZDDP (zinc dialkyldithiophosphate), AW (antiwear), MCL (maximum contaminants limits), PM (particulate matter), PCDF (polychlorinated dibenzofurans), POP (persistent organic pollutants).
- Pollution Definition and Polluted Soils
Pollution can be defined as the introduction of elements, compounds or energy into the environment at levels that impair its functioning or that present an unacceptable risk to humans or other targets that use or are linked to that environment. Historically, pollution of soil has been of limited concern. Humans are at risk from polluted soils through dermal contact, ingestion, consumption of food grown on polluted areas and inhalation of dusts or vapours [1]. Soils may fail to support vegetation because of phytotoxic effects of pollutants or disrupted biological cycling of nutrients. These soils may also affect the hydrosphere compromising the quality of drinking
water resources and threatening the aquatic ecosystems [2].
Pollutants can build up in soils from several sources. The spreading of wastes such as sewage sludge or other biosolids to land can be a problem especially where these wastes have been applied repeatedly over a number of years. Some wastes (e.g. dredgings, pulverised fuel ash or mine spoils) constitute new ‘soil-forming’ materials [3]. Soils may become polluted by atmospheric deposition from traffic, and incinerator or metal smelting emissions over a period of time [4]. Soils may also be polluted through the spillage of liquids such as oil or industrial solvents or through flooding or irrigation with polluted water [5]. Whilst most of the early soil pollution related to metals or other inorganic pollutants, there has been an increasing concern over the last few decades regarding organic contaminants, a reflection of their widespread use in industry as solvents, feedstocks and their presence in industrial wastes [6]. Fuel hydrocarbons, for examples, are major pollutants of soils and aquifers. Combustion processes have led to widespread contamination of soils with polycyclic aromatic hydrocarbons (PAHs) [7].
- Sources and Classifications of Contaminants:
Potential sources of organic contaminants include:
1- Industrial leaks and spills,
2- Leaks from oil and chemical storage tanks,
3- Improper application of pesticides,
4- Careless disposal of cleaners, oil and antifreeze,
5- Improper disposal of household wastes,
6- Landfills and garbage dumps,
7- Leaks from pipeline, and
8- Accidents, spills during transportation (i.e. from real or tank cars).
An even more common type of hydrocarbon spill is leakage from underground storage tanks as shown in Figure (1). Non-aqueous phase liquids (NAPLs) such as petroleum hydrocarbons are a major source of groundwater contamination. Petroleum hydrocarbons are introduced to the environment in a number of ways. The most common sources is leaking underground storage tanks that release millions gallon of NAPLs to groundwater systems annually [8], and during transportation.
The source of contamination is usually classified in space as either a point source or a non-point (diffuse) source. A point source is a contaminant release at one specific location, whereas a non-point source is released over a widespread area. The major point sources are municipal landfills and industrial waste disposal sites, toxic chemicals spewing from pipes at industrial facilities, sewage treatment plants, accidental spills, and leaks of petroleum products of dense industrial organic. Non-point sources include atmospheric deposition, contaminated sediments, and many land activities that generate polluted runoff, such as agriculture and onsite sewage disposal. Atmospheric deposition refers to contaminants entering waters from polluted air. In place contaminants were generated by past activities, such as discontinued industrial discharges, logging, or one-time spills. In-place contaminants often reside in sediments but continue to release pollutants back into the water column. Natural sources refer to natural deposits of salts, gypsum, nutrients, and metals in soils that leach into groundwater.
The source of contamination is also classified in time as either a continuous source or an instantaneous (one time) source. A continuous source is contamination that is released over a long period of time, whereas an instantaneous source is contamination that is released at only one time. The type of contamination source in space and time (that is, point source, non-point source, continuous source, instantaneous source) is important in determining the resulting spatial and temporal distribution of concentrations within a groundwater system. In some cases, the cumulative effects of point sources in proximity with each other can have similar characteristics to one or more non-point sources of contamination. The same sources can also be classified into surface sources (e.g. "spills" which must infiltrate) subsurface sources (e.g. septic tanks and leaking underground storage tanks which already exist in the subsurface). Aquifers can be contaminated from a number of natural processes and human activities.
- Contaminant–Soil Interactions
The contaminant–soil interaction is mainly occurred by (i) sorption, (ii) complexation, and (iii) precipitation [10]. The term sorption is used to indicate the process in which the solutes (ions, molecules, and compounds) are partitioned between the liquid phase and the soil particle interface. Physical adsorption occurs when the contaminants are attracted to the soil constituent's surfaces (from the aqueous solution present inside the pore) because of the unsatisfied charges (attractive forces) of the soil particles. Chemical adsorption occurs by chemical bonding. In specific adsorption, the ions penetrate the coordination shell of the structural atom and are bonded by covalent bonds via O and OH groups to the structural cations. The interaction by complexation and precipitation is occurred by the inorganic contaminants. Organic contaminants like petroleum hydrocarbons are adsorbed physically due to hydrophobic forces on the soil surface. The different physical forms possible for organic contaminants in soil are illustrated in Figure (2).
3.1. Initial Trapping and Distribution of NAPL Residual in Soils
After a hydrophobic organic compounds (HOCs) spill, NAPL migrates towards the water table and may become trapped in unsaturated pores in the vadose zone, held by capillary forces [12]. Capillary forces are a result of interfacial and surface tension between the liquid and gas phases and also between individual phases and the soil media. Near a NAPL spill, a four-phase system exists. The phases include (1) the aquifer matrix, (2) the residual soil water, (3) the NAPL, and (4) the air-filled pore space, or three phases are present within the soil pores: gas, water, and oil (Figure 3). Chemicals are transported through the vadose zone in soils in the gas, aqueous and non-aqueous phase liquids by advection (Figure 4), molecular diffusion (Figure 5), and mechanical dispersion. Chemical advection is often the dominant transport process in soil, and is simply the transport of a chemical with the bulk movement of the phase [13]. Thus, contaminants are transported by primary advection, and their concentration changes as a result of dispersion. Contaminants will also move in response to their chemical kinetic activity from areas of higher concentration to areas of lower concentration and is termed diffusion [14].
It can be generally stated that advective transport and associated mechanical dispersion processes dominate the contaminant transport system in formations of medium-to-high hydraulic conductivity (gravel and sand). In formations of low hydraulic conductivity, including clay liners in waste management facilities, diffusive transport is frequently the controlling mechanism. Requirements for advective transport are: a hydraulic gradient, if not, transport happens by dispersion or diffusion, which are much slower than advection [14].
The region of soil below the water table is called the saturated zone where the soil pores are completely filled with water. A portion of oil which migrates into this region also becomes trapped as residual oil held by capillary forces. This residual can occupy between 10 to 50% of the pore space [17]. When the spill reaches the surface of the water table it will either pool on the surface light non-aqueous phase liquid (LNAPL) or continue to sink downwards through the saturated zone dense non-aqueous phase liquid (DNAPL). Fluctuations in the water table redistribute the pooled NAPL as discontinuous residual droplets, or blobs, trapped in soil pores [12].
During displacement of NAPL by an aqueous phase, capillary forces cause trapping of residual blobs. Residual blobs often occupy single pore bodies, or will span several pores in the soil matrix. Two trapping mechanisms that occur in subsurface systems are called snap-off and by-passing [18]. Snap-off occurs in pores with high aspect ratios [19], which is the ratio of pore body to pore throat diameters. Snap-off is highly dependent on wettability (i.e., contact angle) of the fluid/soil system. As NAPL is displaced from a pore by the aqueous phase wetting fluid, the NAPL is "pinched" by the wetting fluid as it exits the pore. A blob of NAPL is disconnected from the retreating NAPL phase and trapped in the pore body. The snap-off entrapment mechanism promotes formation of small singlet blobs that usually are one or two pore bodies in size. By-passing occurs when water preferentially flows through a small pore rather than an adjacent larger pore. NAPL which may be present in the larger pore thus remains as trapped residual. This occurs because larger capillarity in the smaller pore preferentially draws in the wetting phase (i.e., water). A more comprehensive review of the by-passing entrapment mechanism is given by Wilson et al. [18]. By-passing can extend over several pores creating large complex blobs. Generally, blobs do not extend over 10 pore bodies in length [20].
3.2. Forces Acting on Trapped Residual NAPL Blobs
Capillary forces, buoyancy forces, and viscous forces act on trapped residual blobs. NAPL blobs are held in a soil matrix by capillary forces resulting from interfacial tension (IFT) between water and NAPL phases and wettability of the soil matrix [18]. Capillary forces are proportional to soil pore diameters and increase as pore diameter decreases. Buoyancy forces exerted on trapped residual blobs result from density differences between water and trapped residual. LNAPLs experience buoyancy forces oriented upward. For DNAPLs, buoyancy forces are oriented downward. Viscous forces are hydrodynamic forces due to groundwater flow or the hydraulic gradient applied by extraction wells.
3.3. Long-Term Contamination
The NAPL that remains in the vadose zone is an important source of contamination because it is dissolved by the passing recharge water, and the passing groundwater as the water table rises. Such sources of contamination can last for many years and contaminate large volumes of groundwater. However, in addition to these pathways, contaminants also can be transported through the vadose zone. This transport pathway may spread the contaminants over a much broader area of the aquifer [12]. A considerable amount of hydrocarbon oils can be held in voids in the soil in the form of residual saturation. Because of the low solubility of hydrophobic organic compounds in water, the residual organic phase usually represents a long-term contamination source for soil and groundwater, through the action of rain water or any other source of water, if not removed in time [21]. Owing to the tendency of contaminants to tightly bind or absorb onto the soil particles, subsurface contamination is complex and difficult to treat [22].
Subsurface contamination by the organic compounds is a complex process and difficult to treat due to many reasons like the tendency of adsorption of contaminants onto the soil matrix, low water solubility, and limited rate of mass transfer for biodegradation and so on. As many organic compounds have low solubility in water, so they may leach from the soil for a longer period of time and thus ultimately becomes a continuous source of the soil and groundwater contamination [23]. Understanding the chemistry of the binding of the contaminant and the hydrogeology of the site are very important [24]. Since water solubility of many organic contaminants is the controlling removing mechanism, additives are used to enhance efficiencies.
- Mechanism of Groundwater Pollution by Organics
The important characteristics of the soil that may determine groundwater contamination are texture, permeability and organic matter content. Soil texture is the relative proportions of sand, silt, and clay. Coarse sandy soils allow more water movement by percolation and have less capability to adsorb chemical than clay. Hence, the coarser the texture of a soil the greater is the chance of chemical reaching groundwater. Soil permeability is a measure of how fast water moves downward through the soil. Highly permeable soils have a greater capability to lose chemicals to leaching. Soil organic matter influences soil capability to hold water and to adsorb chemicals. The incorporation of organic matter into a soil will increase the water holding capability and decrease the downward movement of chemicals by leaching.
The procedure selected to contain spills on land will vary with the amount and type of oil spilled, the type of soil and the terrain. Less viscous NAPL and more porous soil will allow greater and more rapid penetration and lateral migration in the soil. Groundwater is very susceptible to contamination, unless protected by a low permeability layer such as clay. The organic contaminants like petroleum hydrocarbons, halogenated organic compounds, or other organic compounds are bind strongly inside the soil matrix and present for long time at the contaminated sites. Many of those organic hydrocarbons are sparingly soluble in water, so they can mix with water during the flow of water through the soil matrix. Ultimately when the contaminated water or refined petroleum which leaks from a storage tank is of sufficient volume, the spill liquid can reach the water table to contaminate the groundwater.
Since hydrocarbon liquids have only limited solubility in water, a relatively small spill can eventually contaminate millions of liters of groundwater. Even, when the surface water is contaminated and that may pass through the soil matrix with low organic content (less sorptive capacity of the organics), the contaminated water may reach the groundwater without purification and contaminate it. Unfortunately, as with the spill of a hydrocarbon liquid, the contamination does not remain localized, since there is also a flow in groundwater in the subsurface, the contaminate water moves away from the contaminated site of the spill.
- Hazardous Waste
A hazardous waste can be defined as a waste with a chemical composition or other properties that make it capable of causing illness, death, or some other harm to humans and other life forms when mismanaged or released into the environment [25]. The common sources of hazardous wastes are: (a) industrial wastes, (b) agricultural wastes, (c) household or municipal waste, and (d) medical wastes. Hazardous wastes may pollute the soil, air, surface water, or groundwater.
Waste control, disposal standards, and requirements are not well established in many countries. As a result, absence of clear and comprehensive regulations makes it difficult to exercise proper control. Presently, initiatives have been taken by many countries via legislation or recommended guidelines and/or standards for neutralization and detoxification of the waste streams to be implemented before discharge. Table (1) shows typical hazardous components in industrial waste streams.
5.1. Waste-Lubricant Oils as a Widespread hazard Contamination
Used oil as an Organic pollutant is considered to be the most widely distributed class of petroleum hydrocarbon result from human activities present in the environment. Heavily contaminated soils are frequently found around industrial plants using petroleum hydrocarbons [27]. The major sources of used oil contamination are old gas stations also oil change stations, industrial locations, leaking underground or aboveground storage tanks, accidental spillage during transferred and often dedicated to agricultural activities. Due to their mobility in soil, they may cause considerable damage also in water intakes or groundwater reservoirs [28].
Lubricating oils, in particular used (waste) lubricating oils, represent one of the most hazardous mainstream categories of environmental pollutants. Whether direct or indirect, their short- and long term impact on soil, waterways, plants, health of animals and humans is substantial if they are handled or disposed of in an uncontrolled manner.
Lubricating oils, including such oils whose primary purpose is other than lubrication (e.g. electric insulation), and lubricating greases contain a base oil component and additives, used to a tailor the lubricant for different applications. Lubricating greases also contain a further compound referred to as the thickener, typically metallic (calcium, aluminium) soap.
The primary purpose of lubricants is to reduce friction between moving parts of various equipment or machinery. The use of lubricants minimises material wear and contributes to improve efficiency of equipment or machinery, and fuel and energy savings. The main categories of lubricants are lubricating oils and lubricating grease. These are used in numerous applications, which can be classified in one of the following two groups:
1- Automotive applications: in marine, air, railway and road transport vehicles, off-road earth moving equipment employed in agriculture, mining, construction works, etc.
2- Industrial applications: in a variety of specific mobile or stationary industrial equipments.
In the context of this study, the terms lubricants and lubricating used oils also refer to as oil, or lay under NAPLs and HOCs categories.
5.2. Environmental Impacts and Risks Related to Lubricants Use
Oil has a tendency to accumulate in the environment. The reduction in additives levels, used to give the oil better performance, is coming under attack, because they cause bio-accumulation problems. It can be seen quite clearly that this type of hydrocarbons is accumulating in a dip in the road, near river mouths, and lakes, and finally in sediments. Bioaccumulation is the concentration (i.e., accumulation) of chemicals from water or food in living organisms. Bioaccumulation is determined by the degree of uptake, distribution, metabolism, and elimination of the chemical in an organism. A typical example is polychlorinated biphenyls (PCBs), which are soluble in lipids, persistent and accumulate in fish [29].
All lubricating based oils are mostly built of carbon (C), hydrogen (H), and lesser extent heteroelements, primarily oxygen (O). Additives are used in order to induce properties which base oil originally lacks, but also to enhance existing desired or reduce/eliminate undesired base oil properties. Lubricant additives can be grouped into three main functional areas [30]: (1) surface protective additives; (2) performance additives; (3) lubricant protective additives. The usual lubricant additives are the so called detergent-dispersant-inhibitor packages, combining detergent/dispersant properties and oxidation/corrosion inhibition, antiwear additives, viscosity index improvers, pour point depressants, and additives inducing extreme pressure (EP) properties. They are based on various organic and organometallic compounds, mostly:
- zinc dialkyldithiophosphate (ZDDP) and borates, as multifunctional additives in lubrication formulations and act as anticorrosion and rust inhibitor, antiwear (AW)/extreme pressure (or temperature) improver EP and antioxidant.
- sulphonates, phenates and salicylates (typically of calcium or magnesium), are surface protective additives (detergent and dispersant).
- olefin copolymers and polymethacrylates are performance additives act as viscosity index improver.
- styrene-butadiene copolymers and styrene-isoprene copolymers, are lubricant protective additives act as antifoamant.
- succinimides, are surface protective additives act as detergent and dispersant.
Taking the base oil component and additives into account, besides hydrocarbons built of organic carbon and hydrogen, new (unused) lubricants contain “hetero” elements, primarily oxygen (O), sulphur (S), nitrogen (N), calcium (Ca), zinc (Zn), phosphorus, boron (B) and chlorine (Cl) in varying concentrations, while past formulations, which sometimes contained very harmful elements such as lead (Pb) and barium (Ba), have been abandoned over the years. Poisoning with these compound are jaundice, vomiting, collapse, and coma. The vomits contain blood and desquamated mucosal lining and collapse. Also these compounds produce skin irritation and acute degeneration of the liver after prolonged exposure. Pathologic findings include acute necrosis of the liver, edema of the kidneys and heart, hematuria in some cases, necrosis of the adrenals, while base oil sometimes used to contain very harmful PAH [31]. It was found that PAHs represent nearly 1% of the volume of used oil. The maximum contaminants limits (MCL) of PAHs in drinking water is 0.0002 mg/l, and their health effects are reproductive difficulties; increased risk of cancer [32].
The term used oil designates the waste product resulting from applications of lubricating oil, whose original characteristics have changed during the use to the extent rendering it unsuitable for further use in the applications it was originally intended for. In terms of chemical composition, along with original ingredients from base oil component and additives, used oil also contains products of oxidation and degradation of the base oil and additives, as well as contaminants induced during the application (e.g. motor oils are often contaminated with traces of fuel, products of fuel combustion and coolant). Used oil often contains approximately 2 to 5 percent additives [33]. In addition to these additives, used oil may include a number of physical and chemical contaminants, including metals, dirt, and solvents [34]. Major pollutants usually present in used oil are various organic acids, polymer resins, metal wear particles (lead, chromium, nickel, cadmium, aluminium, etc.), PAHs, mercaptans, various halogenated compounds and also contain extremely harmful PCB compounds.
Used oil contains 140,000 Btu per gallon [35], a study by Dominguez-Rosado and Pichtel [36] indicates that used motor oil has the following metals concentrations: Zinc: 838 mg/kg, Lead: 110 mg/kg, Cadmium: 9.4 mg/kg, Barium: 4,100 mg/kg and Chromium: Not detected.
Used oils can have numerous substantial, short and long term environmental impacts, if handled or disposed of in an uncontrolled manner. It has been estimated that about 40-50% of lubricating oil is irretrievably lost in use by combustion or leakage (about 20 million tons per year worldwide), and only 25-35% recycled (about 10-15 million tons) leaving about 25% (10 million tons) lost [37]. There is therefore considerable scope for old oil handling improvement. Thus for instance, water containing only 1-2 mg lubricating oil per litre is unsuitable for drinking and dangerous. Oil prevents ingress of oxygen in water, thus diminishing living conditions for numerous species. Pollution of soil and water courses with used oils affects entire flora and fauna. Improper burning of used oils often results in further significant air emissions of pollutants, which may then be inhaled by humans or animals, or end up in soil or waterways once absorbed and carried by raindrops. Finally, once in soil or waterways, harmful substances enter food chains and end up in plants, animals and ultimately humans.
Used oil often improperly disposed of through wastewater drains, intentional spills or burning. Releases with wastewater lead into the septic tanks, open water bodies, or in most favorable conditions to wastewater treatment plant. The latter is not designed for loads that contain high concentrations of used oils, which therefore may affect the entire treatment process. If there is no wastewater treatment plant, the oils enter surface waters, further endangering entire aquatic life and coastal biodiversity. In many cases, the surface water is used for drinking, irrigation thus further endangering human life. In case of oil spills or disposal on land, the soil becomes contaminated, and the contaminants mostly seep further into groundwater. Groundwater, which is essential for recharges of surface water bodies or for water supply uses, is extremely difficult and expensive to remediate. In addition, mobility of contaminants through ground- and surface water is rapidly increased, once the contaminant leaves the soil and enters a water body.
Oil spilled on the ground tends to penetrate top soil rapidly, while heavier oils slowly contaminated soil, as penetration is made difficult by the higher viscosity. Both the viscosity of oily wastes and the characteristics of the ground determine the distance over which the contamination is going to spread. The oil also enters vegetation, either contaminating or entirely killing it. Concerning uncontrolled or improper burning of used oils, it should be stressed that their thermal degradation at temperatures below 1100 ºC can lead to the formation of very poisonous gaseous compounds such as dioxins and furans. Beside this emission released by the practices of oil burning include carbon monoxide (CO), sulphur oxides (Sox), nitrogen oxides (NOx), particulate matter (PM), metals, hydrogen chloride, global warming gases (carbon dioxide – CO2 and methane – CH4), and other organic compounds, all harmful or toxic to the environment. This entire amount ends up in soil, waterways or atmosphere (from which it is further inhaled by animals and humans or ultimately also washed down to soil and waterways). At least 90% of used automotive lubricants (engine and gear oils) are currently burned as a cheap alternative to regular heating fuel. Part of this amount undergoes natural degradation, while a practically unidentifiable portion ends up in plants, animals and humans casing acute or cumulative and chronic health problems. Unfortunately, readily biodegradable lubricants are currently used only in negligible quantities. They are highly toxic and carcinogenic substances; toxicity prevents faster degradation [38].
Paying further attention to the fact that PCB containing used oils are sometimes burned in boilers of central heating systems (with the additional risk of formation of highly toxic polychlorinated dibenzofurans – (PCDF), shortly furans), whether alone or mixed with other types of oils, as well as that there are occasional leaks from transformers, obviously devastating consequences are often not even comprehended. Once in animal or human tissue, PCB as one of persistent organic pollutants (POP) causes disastrous effects. Animals or humans become less fertile, less immune and develop higher rates to cancer, anemia, liver, and kidney or spleen damage, changes in blood or circulatory system problems. It is also believed that PCBs also act as endocrine disrupters by blocking hormone messages [32].
Groundwater receives the input of industrial and urban effluents and thus contains a cocktail of organic contaminants originated in raw materials and everyday products [39], like used oil which contain PAH and/or PCBs, which may be environmentally toxic or recalcitrant. Remediation of soil contaminated with organic hydrocarbons (used oil) is a major environmental concern due to their toxic, mutagenic and carcinogenic properties [40]. Due to their hydrophobicity, used oil has low water solubility and is strongly adsorbed in soils and sediments especially onto terrestrial colloids. Their adsorption onto soils strongly limits the efficiency of remediation techniques [41]. Used oil is particularly difficult to remediate since these contaminants are often mixed with metals i.e. NAPL containing heavy metals [42]. Therefore, biodegradation of used oil is very slow, resulting in their environmental persistence for long periods of time. To reduce the persistence of pollutants and hence preventing their distribution towards subsurface strata, remediation of the contaminated soil during in situ or ex situ treatment must be applied.
Conclusion
The present work provides facts on organic hydrocarbon contaminations to understand its fate in the environment. Whereas, HOCs are the most persistent contaminants in soils and sediments that basically introduced to the ecosystems through leaking or surface spills. HOCs include broad classes of chemicals such as petroleum hydrocarbons, creosotes, and fuel residues. The contaminants can diffuse through pores, adsorb on the surface of the pores, and thus contaminate the groundwater.
Images and Tables
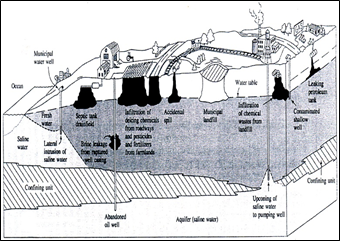
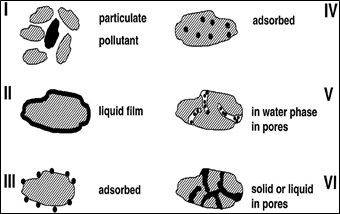
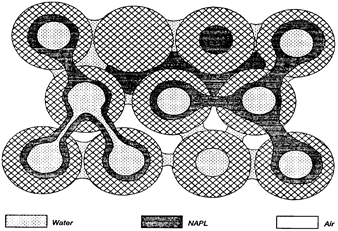
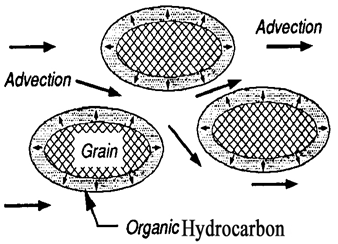
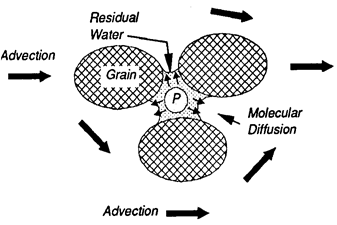
Industry |
Arsenic |
Heavy metal |
Chlor. H'carbons |
Mercury |
Cyanides |
Selenium |
*Misc. Organics |
Chemical |
– |
P |
P |
P |
– |
– |
P |
Electrical & Electronics |
– |
P |
P |
P |
P |
P |
P |
Electroplating metal Ind. |
P |
P |
– |
P |
P |
– |
P |
Leather |
– |
P |
– |
– |
– |
– |
P |
Mining & Metallurgy |
P |
P |
– |
P |
P |
P |
P |
Paint & Dye |
– |
P |
– |
P |
P |
P |
P |
Pesticide |
P |
– |
P |
P |
P |
– |
P |
Pharmaceutical |
P |
– |
– |
P |
– |
– |
P |
Pulp & Paper |
– |
– |
– |
P |
– |
– |
P |
*MSW |
P |
P |
P |
P |
P |
P |
P |
References
Nathanail CP, Earl N. Human health risk assessment: guideline values and magic numbers. In: Hester RE, Harrison RM (eds) Assessment and reclamation of contaminated land. Royal Society of Chemistry, Cambridge, 2001; pp 85?102.
View ArticleScullion J. Remediating polluted soils. Naturwissenschaften. 2006; 93: 51?65. PMid:16468025
View Article PubMed/NCBIWinterhalder K. Environmental degradation and rehabilitation of the landscape around Sudbury, a major mining and smelting area. Environ Rev. 1996; 4: 185?224.
View ArticleHelmisaari HS, Derome J, Fritze H, Nieminen T, Palmgren K, Salemaa M, VanhaMajamaa I. Copper in Scots pine forests around a heavy-metal smelter in south-western Finland. Water Air Soil Pollut. 1995; 85: 1727?1732.
View ArticleSiebe C. Heavy metal availability to plants in soils irrigated with wastewater from Mexico City. Water Sci Technol. 1996; 32: 29?34. 00135-7
View ArticleCollins C, Laturnus F, Nepovim A. Remediation of BTEX and trichloroethene-current knowledge with special emphasis on phytoremediation. Environ Sci Pollut Res. 2002; 9: 86?94.
View ArticlevanBrummelen TC, vanGestel CAM, Verweij RA. Long-term toxicity of five polycyclic aromatic hydrocarbons for the terrestrial isopods Oniscus asellus and Porcellio scaber. Environ Toxicol Chem. 1996; 15: 1199?1210.
View ArticleHall CW, Johnson JA. Limiting Factors in Ground Water Remediation. J Haz Mat. 1992; 32: 215-223. 85093-G
View ArticleKhater AR. Intensive Groundwater Use in the Middle East and North Africa, in Intensive Use of Groundwater Challenges and Opportunities, Llamas R. and Custodio E. (editors), A. A. Balkema Publishers. 2001. PMCid:PMC98467
Tan KH. Inorganic soil constituents. Environmental soil science. New York: Marcel Dekker Inc. 2000; p. 28?79.
Volkering F, Breure AM, Rulkens W.H. Microbiological aspects of surfactant use for biological soil remediation. Biodegradation. 1998; 8: 401?417.
View ArticleMercer JW, Cohen RM. A review of immiscible fluids in the subsurface: Properties, models characterization and remediation, J Contam Hyd. 1990; 6: 107-163. 90043-G
View ArticleLooney B, Falta R. (Eds.). Vadose Zone. Science and Technology Solutions. Battelle Press, Columbus, Richland. 2000; p. 1465.
LaGrega DM, Buckingham PL, Evans JC. Hazardous Waste Management. In: King, P.H., Elianssen, R., (Consulting Eds.) The Environmental Resources Management Group. Mc- Graw-Hill International edn., Civil Engineering Series. Singapore. 1994; 1146p: ISBN 0-07-113454-9.
Schwille F. Dense Chlorinated Solvents in Porous and Fractured Media. Lewis Publishers, Chelsea, ML. 1988.
Palmer CD, Johnson RL. Volatlization and Gas-Phase Transport. In: Site Characterization for Subsurface Remediation. 1991; EPA/625/4-91/026, 259 pp.
Chatzis I, Kuntamukkula MS, Morrow NR. Effect of Capillary Number on the Microstructure of Residual Oil in Strongly Water-Wet Sandstones. SPE Res. Eng. 1988; 3(3): 902-912.
View ArticleWilson JL, Conrad SH, Mason, WR, Peplinski W, Hagan E. Laboratory Investigations of Residual Liquid Organics from Spills, Leaks, and Disposal of Hazardous Wastes in Groundwater. EPA/600/6-90/004, U.S. EPA, U.S. Government Printing Office, Washington, D.C. 1990.
Li Y, Wardlaw NC. The Influence of Critical Pore-Throat Size Ratio on Snap-Off. J. Col. Inter. Sci. 1986; 109(2): 461-472. 90324-3
View ArticleMelrose JC, Brandner CF. Role of Capillary Forces in Determining Microscopic Displacement Efficiency for Oil Recovery by Water flooding. J Can Petrol. Tech. 1974; 54-62. PMid:4532109
PubMed/NCBIMackay D. In: Calabrese EJ, Kostecki PT, Fleis-cher EJ. (Eds.). The Chemistry and Modeling of Soil Contamination with Petroleum, Soils Contaminated by Petroleum: Environmental and Public Health Effects. Wiley, New York. 1985.
Tsai YJ, Chou FC, Cheng S.J. Using tracer technique to study the flow behavior of surfactant foam. J Haz Mat. 2009; 30:166 (2-3), 1232-1237.
Keith LH, Telliard, WA, Priority pollutants: I?A perspective view. Environ Sci Technol. 1979; 13: 416?423.
View ArticleUSEPA (United State Environmental Protection Agency). Treatability studies under CERCLA: an overview. OSWER Directive 9380.3-02FS. 1987.
USEPA (United State Environmental Protection Agency). Performance Monitoring of MNA Remedies for VOCs in Ground Water. EPA/600/R-04/027. 2004.
Yong RN, Mohamed AMO, Warkentin BP. Principles of contaminant transport in soils. Amsterdam, The Netherlands: Elsevier. 1992.
Petitgirard A, Djehiche M, Persello J, Fievet P, Fatin-Rouge N. PAH contaminated soil remediation by reusing an aqueous solution of cyclodextrins. Chemosphere. 2009; 75: 714?718. PMid:19251300
View Article PubMed/NCBIGallego JLR, Loredo J, Llamas JF, V?zquez, F, S?nchez J. Bioremediation of diesel-contaminated soils: evaluation of potential in situ techniques by study of bacterial degradation. Biodegradation. 2001; 12: 325?335. PMid:11995826
View Article PubMed/NCBIDroy BF, Randies SJ. In synthetic lubricants and high-performance functional fluids, Eds., L.R. Rudnick and R.L. Shubkin, 2nd Edition (Revised and Expanded), Marcel Dekker, New York, Chapter 34. 1999.
Pirro DM, Wessol AA. (Lubrication Fundamentals, Second Edition, Marcel Dekker, New York. 2001.
Dreisbach RH. Handbook of Poisoning: Prevention, Diagnosis and Treatment, Thirteenth Edition. Drawer L, las altois, Califorinia, USA. 704 pp. 1980.
Paria S. Surfactant-enhanced remediation of organic contaminated soil and water. Advances in Colloid and Interface Science. 2008; 138: 24?58. PMid:18154747
View Article PubMed/NCBIDOE, (United States Department of Energy). Used Oil Re-refining Study to Address Energy Policy Act of 2005 Section 1838. 2006.
USEPA (United States Environmental Protection Agency). Code of Federal Regulations: Standards for the Management of Used Oil. accessed August 28, 2008 at .
View ArticleUSEPA (United States Environmental Protection Agency). Managing Used Oil: Advice for Small Businesses. accessed August 19, 2008 at .
View ArticleDominguez-Rosado E, Pichtel J. Chemical Characterization of Fresh, Used, and Weathered Motor Oil via GC/MS, NMR, and FTIR Techniques. Proceedings of the Indiana Academy of Sciences. 2003; 12(2): 109-116.
Lansdown AR. Lubrication and Lubricant Selection, A Practical Guide, Third Edition. Professional Engineering Publishing Limited, London and Bury St Edmunds, UK. 285 pp. 2004.
Colombani, N., Mastrocicco, M., Gargini, A., Davis, G.B., Prommer, H., Modelling the fate of styrene in a mixed petroleum hydrocarbon plume. Journal of Contaminant Hydrology. 2009; 105: 38?55. PMid:19117639
View Article PubMed/NCBIMarttinen SK, Kettunen RH, Rintala JA. Occurrence and removal of organic pollutants in sewages and landfill leachates. Sci Total Environ. 2003; 301: 1-12. 00302-9
View ArticleBamforth SM, Singleton I. Bioremediation of polycyclic aromatic hydrocarbons: current knowledge and future directions. J Chem Technol Biot. 2005; 80: 723?736.
View ArticleTiehm A, Stieber M, Werner P, Frimmel F. Surfactant-enhanced mobilization and biodegradation of polycyclic aromatic hydrocarbons in manufactured gas plant soil. Env Sci Technol. 1997; 31: 2570?2576.
View ArticlePankow JF, Cherry JA. Dense Chlorinated Solvents and other DNAPLs in Groundwater: History, Behavior, and Remediation. Waterloo Press, Ontario. 1996; pp. 337-506.